Scientists Can Now Revive The Wooly Mammoth
Here's how
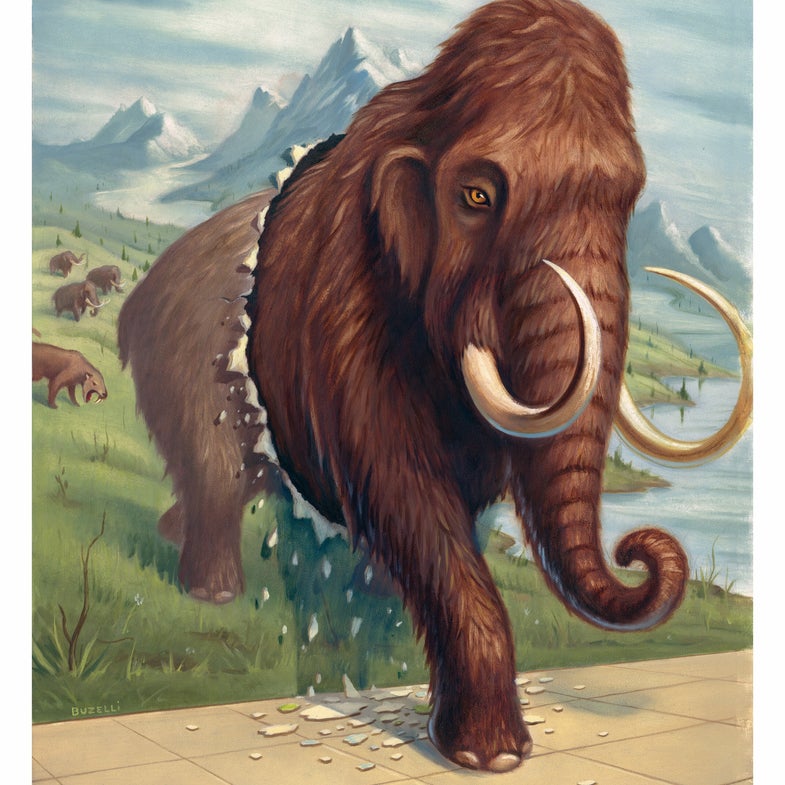
The first use of the word “de-extinction” was, as far as I can tell, in science fiction. In his 1979 book The Source of Magic, Piers Anthony describes an explorer who suddenly finds himself in the presence of cats, which, until that moment, he had believed to be an extinct species. Anthony writes, “[The explorer] just stood there and stared at this abrupt de-extinction, unable to formulate a durable opinion.”
I imagine this is precisely how many of us might react to our first encounter with a living version of something we thought was extinct. The idea that de-extinction may actually be possible—that science could advance to the point where extinction is no longer forever—is both exhilarating and terrifying, even to me. I am a biologist. I teach classes and run a research laboratory at the University of California at Santa Cruz. My lab specializes in a field of biology called ancient DNA. We and other scientists working in this field develop tools to isolate DNA sequences from bones, teeth, hair, seeds, and other tissues of organisms that used to be alive. We then use those sequences to study ancient populations. The DNA we extract from these remains is largely in terrible condition, which is not surprising given that it can be as old as 700,000 years. During my career, I have studied DNA from an assortment of extinct animals, including dodos, giant bears, steppe bison, North American camels, and saber-toothed cats. By piecing together the DNA sequences that make up these genomes, we can learn nearly everything about an individual animal’s evolutionary history: how and when the species to which it belonged first evolved, how the population in which it lived fared as the climate changed during the ice ages, and how the physical appearance and behaviors that defined it were shaped by the environment in which it lived. I am fascinated and often amazed by what we can learn about the past simply by grinding up a piece of bone and extracting DNA from it. Yet, regardless of how excited I feel about our latest results, the most common question about them I receive is, “Does this mean that we can clone a mammoth?” Always the mammoth. The problem with this question is that it assumes that, because we can learn the DNA sequence of an extinct species, we can use that sequence to create an identical clone. Unfortunately, this is far from true. To clone Dolly the sheep in 1996, scientists at the Roslin Institute, which is part of the University of Edinburgh in Scotland, removed a small piece of mammary tissue that contained living cells from an adult ewe. They used the DNA in these cells to create an identical copy of the ewe. This process is called somatic cell nuclear transfer, or, more simply, nuclear transfer. For species that have been extinct for a long time, however—the passenger pigeon, the dodo, the mammoth—cloning by nuclear transfer not a viable option. It requires intact cells. No such cells have ever been found in the remains of extinct species recovered from the frozen tundra. Degradation of cellular DNA begins immediately after death. Plant and animal cells contain enzymes whose job it is to break down DNA. These enzymes, called nucleases, are found in cells, tears, saliva, sweat, and even on the tips of our fingers. Nucleases are essential while we’re alive. They destroy invading pathogens before they can do any damage. They remove damaged DNA so that our cells can fix what’s broken. And, after our cells die, they break down the DNA in those dead cells so that our bodies can more efficiently get rid of them. In the lab, we stop nucleases from degrading away the DNA we’re trying to isolate, either by dropping a fresh sample into a solution of chemical inhibitors or by subjecting it to rapid freezing. The Arctic is a cold place, but it can’t freeze something as large as a woolly mammoth quickly enough to protect its DNA from decay.
The other path to creating a living organism is eerily reminiscent of the movie Jurassic park.
Besides nuclear transfer, the other path to creating a living organism is eerily reminiscent of the movie Jurassic Park. As is likely to be true in real-life de-extinction projects, Jurassic Park scientists were able to recover only parts of the dinosaur genome—in their case, from mosquito blood preserved in amber. When they came across gaps in the dinosaur genome, they used frog DNA to complete the sequence. Unfortunately, they couldn’t know beforehand which bits of DNA would help make a dinosaur look and act like a dinosaur and which bits were junk. We can assume these fictional scientists were hoping that the holes they were filling were mostly in the junk-containing regions. But, of course, they were wrong, and some of that frog DNA let the unextinct dinos switch sexes miraculously, leading to disaster and $1 billion in global box-office earnings. In real-life de-extinction science, the plan is to determine which parts of the genome are important in making the extinct species look and act the way it did. We would then find the corresponding parts of the genome of a close living relative, cut out key sequences, and replace them with versions from the extinct species. That’s easier said than done.
The common ancestor of mammoths, Asian elephants, and African elephants lived about six million years ago. This means the mammoth spent many millions of years evolving separately. Some of the hardest parts of the mammoth’s genome to assemble will be those that changed in mammoths since they diverged from elephants. For the purposes of de-extinction, these sequences will likely be the most critical to get right. Fortunately, we don’t have to clone a mammoth to resurrect mammoth traits or behaviors. We could, for example, learn the DNA sequence that codes for mammoth-like hairiness and then change the genome of a living elephant to make a hairier one. Obviously, resurrecting a mammoth trait is not the same thing as resurrecting a mammoth, but it is a step in that direction. Scientists know much more today than they did even a decade ago about how to manipulate cells, sequence the genomes of extinct species, and engineer the genomes of living ones. These three technologies pave the way for the most likely de-extinction scenario, or at least the first phase: the creation of a healthy individual. Here’s how that might work. First we find a well-preserved bone from which we can sequence the complete genome of an extinct species, such as a woolly mammoth. Then we study that genome, comparing it with those of living relatives. The mammoth’s closest living relative is the Asian elephant, so that is where we will start. We identify differences between the elephant’s genome and the mammoth’s, and we design experiments to tweak the elephant genome, changing a few DNA bases at a time, until it looks more mammoth-like. Then we take a cell that contains one of these new, mammoth-like genomes and allow it to develop into an embryo. Finally, we implant this embryo into a female elephant, and about two years later, that elephant gives birth to a baby mammoth. Scientists have already sequenced most of the mammoth genome, which was pieced together from fragments of DNA extracted from mammoth bones. Researchers in George Church’s lab at Harvard University have also taken the next step. They used a new genome-editing tool to splice the DNA that codes for 14 mammoth characteristics—among them, denser hair, thicker layers of fat, and blood cells that could transport oxygen more efficiently at cold temperatures—into an elephant genome. It is not yet possible to grow these cells into a whole organism, but the Harvard team is working to develop that technology as well. If successful, the embryo, whose genome contains some very tiny portion of mammoth DNA, could eventually develop into a living, breathing animal. But what would be the end product of this experiment? Is making an elephant whose genome contains a few mammoth parts the same thing as making a mammoth? After all, an animal is more than a simple string of As, Cs, Gs, and Ts—the letters that represent the nucleotide bases that make up DNA. Today, we don’t fully understand the complexities of how we get from simply stringing those letters together in the correct order to making an organism that looks and acts like the real thing. That will involve much more than merely finding a well-preserved bone and using it to sequence a genome.
When I imagine a successful de-extinction, I don’t imagine an Asian elephant giving birth to a slightly hairier elephant under the close scrutiny of veterinarians and excited scientists. I don’t imagine the spectacle of this exotic creature in a zoo enclosure, on display for the gawking eyes of children who’d prefer to see a T. rex or Archaeopteryx anyway. What I do imagine is the perfect arctic scene, where mammoth (or mammoth-like) families graze the steppe tundra, sharing the frozen landscape with herds of caribou, horses, and reindeer—a landscape in which mammoths are free to roam, rut, and reproduce without the need for human intervention and without fear of re-extinction. This constitutes the second phase of de-extinction, which builds on the successful creation of an individual to produce and eventually release an entire population into the wild. In my mind, de-extinction cannot be successful without this second phase. That idyllic arctic scene might be in our future. But first, science has some catching up to do with the movies. Though we have sequenced nearly the entire mammoth genome, that work is not yet complete. We are also a long way from understanding precisely which bits of that sequence are important to making a mammoth look and act like one. This makes it hard to know where to begin and nearly impossible to guess how much work might be in store for us. Another problem is that some major differences between species or individuals, such as when a particular gene is turned on during development, are inherited epigenetically. That means that the instructions for these differences are not coded into the DNA but are determined by the environment in which the animal lives. What if that environment is a captive breeding facility? Baby mammoths, like baby elephants, ate their mother’s feces to establish a microbial community capable of breaking down the food they consumed. Will it be necessary to reconstruct mammoth gut microbes? A baby mammoth will eventually need a large, open space where it can roam freely but also be safe from poaching and other dangers. This will likely require a new form of international cooperation and coordination. My goal is not to argue that de-extinction will not and should never happen. In fact, I’m nearly certain that someone will claim to have achieved de-extinction within the next several years. I will argue, however, for a high standard by which to accept this claim. Should de-extinction be declared a success if a single mammoth gene is inserted into a developing elephant embryo and that developing elephant survives to become an adult? Purists may say no, but I would want to know how inserting that mammoth DNA changed the elephant. What if a somewhat hirsute elephant is born with a cold-temperature tolerance exceeding that of every living elephant? And what if that elephant not only looks more like a mammoth but is also capable of reproducing and sustaining a population where mammoths once lived? While others will undoubtedly have different thresholds for declaring de-extinction a success, I argue that this—the birth of an animal that is capable, thanks to resurrected mammoth DNA, of living where a mammoth once lived and acting, within that environment, like a mammoth would have acted—is a successful de-extinction, even if the genome of this animal is decidedly more elephant-like. De-extinction is a process that allows us to actively create a future that is better than today, not just one that is less bad than we anticipate. It is not important that we cannot bring back a creature that is 100 percent mammoth or 100 percent passenger pigeon. What matters is that—today—we can tweak an elephant cell so that it expresses a mammoth gene. In a few years, those mammoth genes may be making proteins in living elephants, and the elephants made up of those cells might, as a consequence, no longer be isolated to pockets of declining habitat in Africa and Asia. Instead, they will be free to wander the open spaces of Siberia, Alaska, and Northern Europe, restoring to these places all the benefits of a large dynamic herbivore that have been missing for around 8,000 years. Large herbivores knock down trees and trample bushes, for example, and transport seeds and nutrients over long distances. By removing snow, mammoths—or rather, cold- tolerant Asian elephants—may also expose the permafrost to the bitter cold of Siberian winters. This would lower the temperature of the soil and slow the release of greenhouse gases trapped within it. De-extinction is a markedly different approach to planning for and coping with future environmental change than any other strategy that we, as a society, have devised. It will reframe our possibilities.
A Model Specimen
not all clones require DNA. ancient creatures can be programmed to roam the earth
This article was originally published in the June 2015 issue of Popular Science.
Life AFter Death
text tk
–Shannon
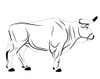
Aurochs
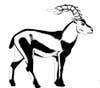
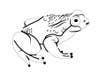
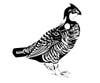
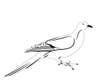