

Look up. Somewhere beyond our solar system, where it’s well below zero, pitch-dark, and the next-nearest star is a 400-century ride away, an electrical charge sparks a radio signal. The blip is faint, some 22 watts, no more power than a typical refrigerator bulb needs. The source is Voyager I. Its 12-foot antenna is calling home from the blackness.
Twenty-some hours later, after an epic interstellar journey, this rippling wave will reach Earth. By the time the ping gets here, its strength has dramatically diminished—down to about 0.1 billion-billionth of a watt. The signal’s trip across our solar system is over, but its voyage has just begun. The challenge now is greater than crossing our corner of the galaxy; it is to hear and make sense of the information within the message, the most distant whisper of our own creation.
Capturing this tiny sliver of nearly nothing takes highly trained and technical ears. Several of them. For Voyagers I and II, they take the shape of three 21-story-tall dishes—each with a diameter of 230 feet and a heft of nearly 3,000 tons—positioned evenly across the globe. Constructed specifically for deep-space listening, they turn skyward, ready to receive the probes’ daily status reports.
One of the dishes, dubbed DSS-14, looms over a lonely patch of Southern California’s Mojave Desert, approximately 60 miles from the nearest highway. It nestles in a small valley, between low craggy mountains—the remnants of long-dead volcanoes. To get anywhere near it, you pass through two layers of security gates at Fort Irwin military base. An extensive briefing introduces you to the neighbors, which include unexploded ordnance, three species of rattlesnake, camel spiders, scorpions, and packs of feral donkeys, which have a yen to gallop up and bite a chunk out of an unsuspecting visitor just for the hell of it.
DSS-14 appears around a bend in the road, standing like a sentinel, casting a long, warped shadow on the bright desert floor. Overhead, turkey vultures ride the wind by its rim. All is silent, apart from the gusts and the crunch, crunch, crunch of footsteps on the sand. But there is another sound too, somewhere far beyond all human hearing, mixed in with the hum of the rest of the universe.
The Fort Irwin-based complex—which NASA’s Jet Propulsion Laboratory named Goldstone after a long-abandoned mining town—houses about a dozen smaller dishes too, the first of which went online in 1958. (A decommissioned Apollo antenna still haunts the scrubby ground.) There are similar fields in the Australian bush, outside Canberra, and in Robledo de Chavela, near Madrid, which were established in 1965. DSS-14 and its international copies went up in the 1960s and ’70s. Since the Mercury IV mission in 1964, these three arrays have linked us with every craft we’ve sent above low Earth orbit.
The positioning of the gargantuan ears is key. The three sites are spaced by longitude (120 degrees apart for full 360 coverage) and united in proximity. Proximity to approximately nothing. Isolation prevents the high-powered space signals and earthly communications—like chatter from air-traffic control—from interfering with one another.
The three facilities and their antennas comprise the Deep Space Network, a program run out of JPL headquarters near Pasadena, California. The system constantly listens for pings from and sends commands to about 40 probes, spacecraft, satellites, and rovers. Some are as close as the moon. Others are way afield, like Juno whizzing around Jupiter, and New Horizons, which in mid-2015 buzzed Pluto. And, of course, Voyagers I and II, both of which launched in 1977 to study Jupiter and Saturn. They’re the oldest and farthest-flung missions NASA or JPL—or anyone—oversees.
Downloads from the two Voyagers deliver insights about the rarefied world of interstellar space: observations about low-energy charged particles, magnetic fields, and the plasma that accounts for the majority of our universe. The data rides the waveform as strings of ones and zeros, at a rate of 160 bits per second (that’s one-fifteenth the info of the slowest fax-machine connection). Studying it has helped physicists do things like outline the contours of the heliosphere—the magnetic bubble that surrounds our solar system—and determine the speed of the sun’s wind.
At a time when NASA is experimenting with faster, denser light-based communication systems, it’s easy to assume radio will fade away. Yet one will never eclipse the other. As the Voyager probes push the technology to its outer limits, they provide a reminder of all the unique insights radio waves collect along their flight path. The noise the signals pick up as they ripple past planets, moons, and asteroids provides a window onto our cosmic neighborhood. In some instances, the static is as valuable as the message itself.

Embedded in the floor of a room in the Jet Propulsion Lab’s campus is a plaque that reads The Center of the Universe. Every signal from every object we send out into the solar system travels into and out of this facility. The so-called Dark Room—whose dimness brightens in the glow of dozens of monitors—has been staffed 24/7 since the earliest days of the Deep Space Network. Not much can shut down operations here. Not rain, not most earthquakes, not even a fire. When a blaze did break out several years ago, the engineers tended the terminals remotely through the smoke, lest they miss even one call from space.
At the moment, huddled around a pair of screens, two bearded men stare at a string of numbers and color-coded lines. It’s a downlink, coming from the probe Juno, which has been orbiting Jupiter since 2016. Mike Levesque, who manages the network’s operations and relevant Dark Room activities, stands nearby, watching, explaining the process. “Those are the data systems operators,” he says, nodding at the two bearded men. “It’s their job to extract the spacecraft information”—the temperature, the fuel, what’s turned on, what’s turned off—“and send it to mission support.” On Voyager I, for instance, of 160 bits, only about 10 are relevant to the goings-on aboard the craft.
The rest of the packets of data travel elsewhere, mostly to scientists, not engineers. The former care about what instruments tell us about the space around the probe, rather than the probe itself.
The two men in front of the screens run programs that clean up all the ones and zeros. But sometimes they save the noise because the interference is also of interest. As a signal propagates through any medium, getting pummeled by an atmosphere or a gravitational field, resulting changes in the wave reveal truths about space. “When a craft is moving through something interesting, noise data is going to be what we want,” Levesque says. In those moments, “the noise in the signal turns out to be science.”
When that happens, the data goes to Kamal Oudrhiri, who heads JPL’s Planetary Radar & Radio Sciences Group. To understand his field, he explains, it helps to imagine a school bus full of children. The driver’s singular objective is to safely deliver all the kids. But what if you didn’t care about the rugrats at all? What if, instead, what really interested you was the bus?
The schoolchildren in Oudrhiri’s analogy are the data, carried by the signal. The signal is the bus. Flight engineers and the staffers tending Mission Control care about the data, the same way almost everyone cares about the kids. But radio scientists find the vehicle itself more interesting because it’s filled with noise.
If you study the bus carefully, you can figure out what it went through as it wound toward its destination. Marks, imperfections—the ugly, misshapen bits—tell you about the journey: not just the road traveled, but the other vehicles, the weather, the traffic along the route. Folks like Oudrhiri scrutinize these myriad faults on a scale, oh, about the size of the universe.
Many of the earliest radio-science experiments were unintentional. Back in 1971, when the Mariner 9 probe passed Mars, its signal traveled through the Red Planet’s atmosphere, which collided with and changed the wave. “People in telecom saw it as an interference, but others saw that if you studied the interference, you could determine the density, the pressure, even the temperature of the atmosphere on Mars,” Oudrhiri continues. “That was the start of radio science.”

Since then, looking carefully at space-borne noise has deepened our understanding of the solar system. Disturbances in the Cassini probe’s transmissions, for instance, helped reveal that Saturn’s colorful rings formed much later than the planet itself did—10 million to 100 million years ago, versus 4.5 billion. NASA’s GRAIL lunar mission in 2012 involved two craft pinging radio waves back and forth to learn about the interior of the moon; inspecting how gravity fields interfered with the transmissions helped prove that most of the orbiter’s crust isn’t as dense as we previously thought.
Oudrhiri loves radio science for its simplicity. A signal is a wave with amplitude (the highs and lows), phase (the pattern of those peaks and troughs), and frequency (the number of dips and spikes in a given span). Distortion in these features is easy to spot. If you know approximately how the ripples should appear, you know when they’ve changed. It’s like a smoke signal blowing away before you can make out the pattern, which tips you off to an unfelt breeze.
A Voyager probe’s cosmic hum always contains one vital piece of radio science. As either craft continues its 38,000-mile-per-hour flight farther into deep space, an acoustic phenomenon known as the Doppler effect slightly stretches its signal’s wavelength—the same way the tone in a siren’s wail warps as an ambulance speeds by. The change tells the ground crew how far Voyager has flown between its daily check-in and the 20-ish hours it takes the call to reach us. It also helps them continue charting the interstellar pioneer’s course. If they know where the thing’s going, they know where to turn those giant antennas to listen for it again.
Now that each probe has completed its primary mission, the new goal is “how can we stretch it out and stretch it out—how long can we make it go?” says Suzanne Dodd, project manager for Voyager and head of JPL’s Interplanetary Network Directorate.
Delivering commands to these extra-solar explorers—working to slow our waning opportunity for deep-space insight—is mainly about managing the probes’ power. Every redundant system on board has, at this point, been turned off. That means both craft are generating very little heat in the extreme interstellar cold, so the hydrazine propellant in the fuel lines might freeze. Mission Control cycles through systems, seeing what might be worth keeping alive for the sole purpose of warming the lines. It’s a patch job, on some of the oldest computers still going.
Voyager mission control sits a couple of miles outside the JPL campus, in a cinder-block building with no signs and high windows—a blink-and-you-miss-it edifice plopped behind a wall of foliage. There’s a McDonald’s next door. This is where a team of 12 keeps the most distant objects humans have ever made alive, steered, nursed, and cajoled onward into the cosmos.
There, folks want to hear the signal, not the noise. Spacecraft systems engineer Fernando Peralta cares deeply about the messages Voyagers I and II send home—about the kids on Oudrhiri’s proverbial bus. Anything that isn’t perfect, any fuzz, troubles him. “When we get the signal, and I see it’s wavy, I think, Why is it moving up and down? It might be the general health of the spacecraft—or it’s just the fact that you have a cloudy day, or a windy day. But to us, too much noise is a catastrophe.”
A garbled ripple also risks losing views of a soundscape only these craft can provide. Voyager I has a digital eight-track on board to record plasma waves, fluctuating ions and electrons that create a sort of oceanic current beyond the bounds of the solar system. The deck is still powered up (in part because it gives off enough heat to keep the fuel lines thawed) and captures 48 seconds of ambient rumbles three times a week. When Voyager I dumps the data, all the active antennas in either California or Spain spend at least four hours pulling down the wavy, ethereal roar of blooping static from the reaches of deep space.
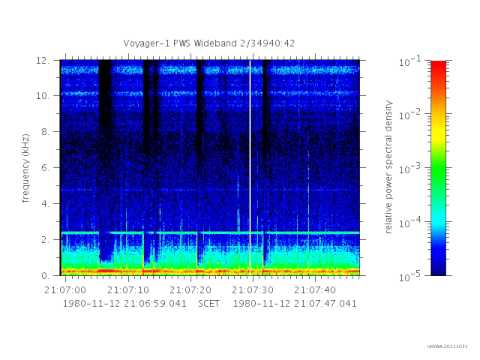
Peralta walks through a huddle of cubicles. Above him, a small sign suspended from the ceiling reads Mission Control. Modern computers flank a microfilm reader, where the team consults old blueprints. He’s overseen, at this point, the shutdown of many systems on both Voyager probes. Each day that he’s able to come in to find that the pair has called home again, it feels like an extra day. “This is such a special location in space, one we aren’t likely to return to in my lifetime or, likely, any of our lifetimes,” he says. “The data is very, very valuable. It’s precious to us. It keeps us connected.”
Soon—a few months, a few years—that connection will cease. The heat will diminish enough on Voyager I or II that a fuel line will freeze, the propellant will no longer be able to reach the thruster, and the craft won’t be able to adjust its flight path ever so slightly, steer its antenna toward Earth, and say hello. Its messages might still cascade through space, but we won’t be able to catch and decipher them. Project manager Dodd is matter-of-fact when describing it: “You’d lose the signal. And that will be that.”
Even before Voyager II runs out of gas, the mission team is preparing for a period of disconnection. Because of the craft’s trajectory away from Earth, which dips below the plane of the solar system, only the dish Down Under can hear it. That antenna won’t transmit for nearly a year as NASA outfits it with test arrays of mirrors and optical sensors for an upcoming push to supplement radio-based communications with light. In letting the probe float on its own for 10 months, the agency is making a strategic sacrifice: stop sending commands to Voyager II and its radio-only antenna in order to allow a new generation of craft to phone home long after the older probes go silent.
For decades, NASA has been experimenting with augmenting its network this way—using pulses of laser light, which can ferry exponentially more data more quickly and to smaller sets of ears. There are a couple of reasons for this. First, space is getting crowded, and all those signals are cluttering radio comms and making scheduling on the Deep Space Network a complex and time-consuming task. Second, as we continue to prospect places like Mars, we will need to dump far more video in far less time. When it launches in 2022, NASA’s asteroid- studying Psyche probe will be among the first to get an optical-comms setup.
Light has its limits though. Clouds, for example, can bend and block it, whereas radio waves can wiggle right through most atmospheric conditions. The systems are also quite cheap, relatively speaking. “You always pay a heavy cost for mass in space,” radio scientist Oudrhiri says. The tech his work relies on rides on the backs of existing equipment, all of which have been around since our earliest space explorations, from Echo balloons to Apollo to today.
Deep-space communication via radio waves will never go away because it’s straightforward and flat-out works. “People often think of very complex solutions to complex problems,” Oudrhiri says. “But so often the solution can be found in the most simplistic thing: Just look at all we’ve learned from paying attention to how the signal varies.”
Quite a lot, it turns out: the density of the moon, the age of Saturn’s rings, the bounds of the solar system. The signal and its noise help us understand our place in the universe—the roads and weather around the lonely blue rock that is Earth.
This story originally published in the Noise issue of Popular Science.