What comes after the James Webb Space Telescope? Some astronomers want LIFE.
The Large Interferometer for Exoplanets could chase new exoplanets and galaxies with a fleet of tools—if it ever gets off the ground.
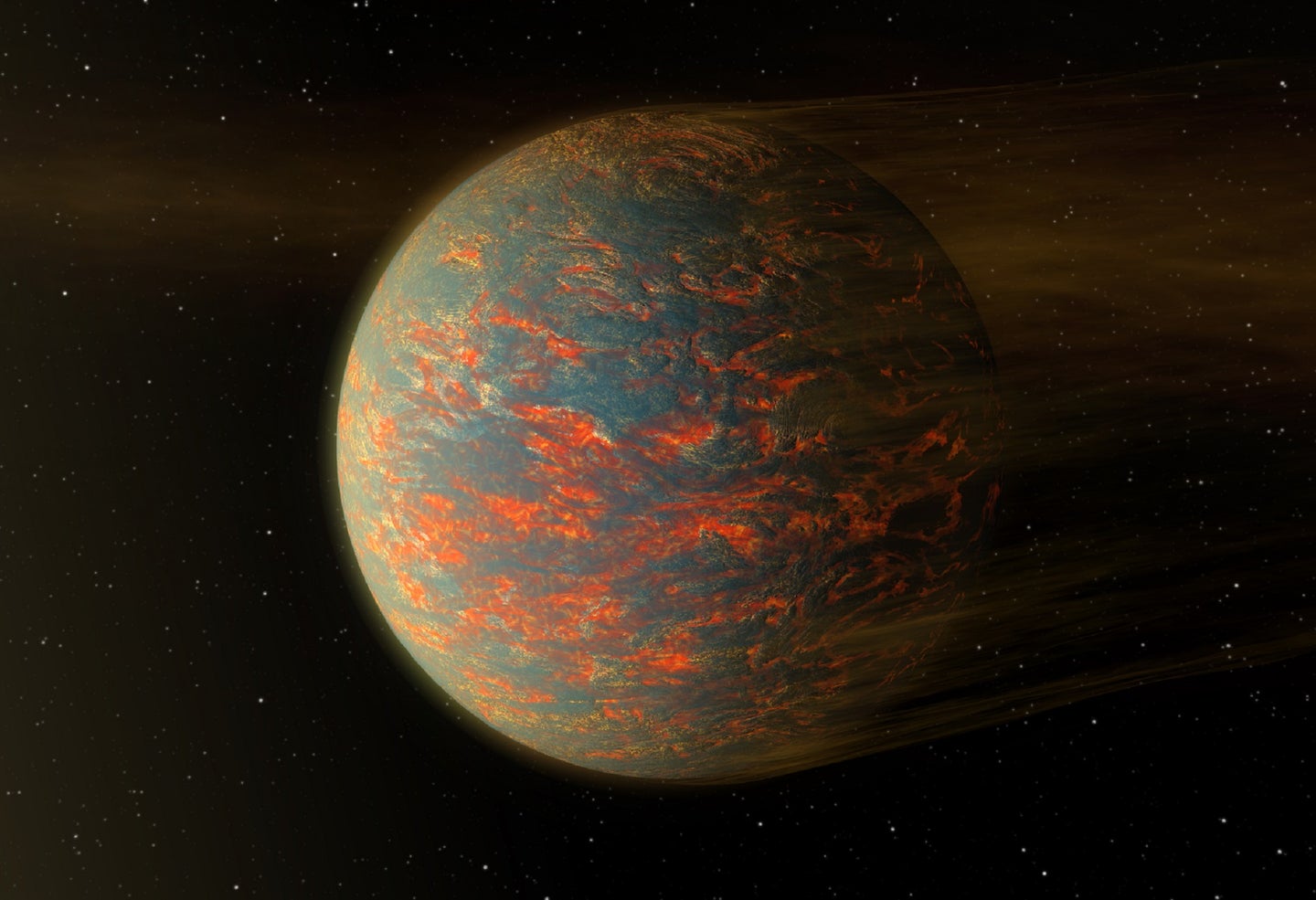
As the largest and most complicated human-made contraption ever delivered to the cosmos, the James Webb Space Telescope (JWST) harrowed astronomers with its launch last year. The telescope has unprecedented sensitivity to peer deeper into the inky universe and resolve distant objects. But the next generation of further-seeing telescopes may not necessarily have to beat JWST’s heft to top its vision.
“If you want to keep getting a better angular resolution, then either you have to build bigger and bigger telescopes—or you have to switch to interferometry,” says Scott Gaudi, an astronomer and exoplanet hunter at the Ohio State University.
Nulling interferometry is an observation technique that gathers data on astronomical objects by mixing light from multiple simultaneous views of the same target. Nulling refers to how this light can be combined to block out the overwhelming background from an object, such as a star, to enhance the signals originating from a much fainter target, such as the orbiting planet that basks under the star’s glare. The technique gets around one of the thorniest challenges for exoplanetary observations: the contrast problem. Compared to other rival technologies, nulling interferometry may be the best candidate to dim down starlight by ten billion times or more—enough to reveal an Earth-sized planet’s lurking presence. And these rocky bodies are prime candidates for housing extraterrestrial life.
[Related: There is no Planet B]
For higher sensitivity and better resolution, each collector of light needs to be spaced out hundreds of meters from each other. Here’s the innovative part: Instead of deploying an unwieldy contraption spoked with multiple detectors, another solution is to remove any bridging struts in the deadspace between the collectors and rely on formation flying. The advantages of autonomous formation flyers are profound—these detectors can move outward or inward to home in on different targets, swivel around, and merry-go-round the central collector to modulate the astronomical signals they receive.
“Interferometry just keeps coming up—over and over and over,” says Gaudi. “I think that it’s very much in the future.”
While a space nulling interferometer isn’t going to be deployed in the next decades, one effort led by Sascha Quanz, an astrophysicist at ETH Zurich, Switzerland, is pushing nulling interferometry from the drawing board to reality. The mission he champions makes no secret of its purpose: LIFE, which also stands for the Large Interferometer for Exoplanets, will scour the cosmos for Earth-like and potentially habitable exoplanets. His team’s proposed infrared observatory involves five individual spacecraft flying in sync to achieve the resolution of a telescope with a 1,970-feet-wide primary mirror. (In contrast, the primary mirror of the world’s largest space observatory, JWST, is 21 feet wide.) Last year, the European Space Agency (ESA) selected exoplanet hunting as one of its three major mission themes in the coming decades, for which LIFE could be a major contender to carry it through. LIFE’s concept is picking up steam, but it’s not the first time nulling interferometry in space has garnered interest—decades ago, the idea was foiled by technological and financial hurdles that made extraterrestrial nulling interferometry practically infeasible. In the decades since, technology improvement has brought nulling interferometry closer to reality than ever before. LIFE could be the mission to pull off nulling interferometry’s redemption story.
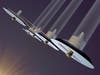
The origins of LIFE
Before the 2000s, exoplanet discoveries were far and few in between, because humankind simply didn’t have the tools to seek them out. But not for the lack of trying—scientists floated around ideas for exoplanet hunters that were considered far too ambitious for their time.
Nulling interferometry was first proposed by Stanford University electrical engineer Ronald Bracewell in 1978. Later, NASA and ESA independently picked up the concept by giving the green light to the Terrestrial Planet Finder Interferometer (TPFI) and Darwin missions in 2002 and 1993 respectively. To the chagrin of the scientific community, budget constraints led to the cancellation of TPFI in 2007. In the same year, ESA scrapped Darwin. In both missions, technology and exoplanet knowledge back then were decidedly insufficient to justify the gargantuan financial costs. Both space agencies shelved the ideas, consigning them to the dusty depths of history as dead-end projects.
Back then, exoplanet hunting was a risky venture. Perhaps the investment in a radical new technology such a space nulling interferometry wasn’t worth it if there weren’t many new worlds to unveil in the first place.
All that changed when a new kid arrived on the block: Kepler.
The nifty space telescope used a method called “transits” to root out covert exoplanets. It stared at a star long enough for an exoplanet to orbit around it several times; periodic blips in the starlight would hint at a possible exoplanet that was transiting in front of—essentially photobombing—the star. To carry out the transit method, this steadfast stargazer surveyed the same patch of sky throughout its heyday.
Kepler first set up shop in 2009 and detected its first previously unknown exoplanet a year later. Hundreds of exoplanets had been discovered in the decade before Kepler’s debut. Nevertheless, the number was too low for scientists to know whether exoplanets, let alone habitable ones, were common or rare—until the Kepler mission ushered in a gold rush of exoplanetary discovery. As many stars as there are strewn across galaxies, even more exoplanets populate the universe, scientists realized. Among the more than 5,000 currently known exoplanets, Kepler scoped out more than half of them in the nine years it silently sentineled the night sky.
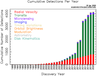
Despite Kepler’s productivity, the transit technique comes with its own limitations: It’s essentially a long waiting game for exoplanets to complete several round trips around their stars. As such, the steadfast stargazer can only afford to stare at the same sliver of sky for several years at a time. Imagine the bonanza of exoplanets scientists could uncover, says Quanz, if humanity had an observatory that could behold the entire cosmos and track exoplanets in real time without having to wait for them to complete their orbits.
Quanz and his team took the statistics from Kepler’s productivity and simulated the rate of return of a hypothetical mission like LIFE that can detect exoplanets directly. “We simply wanted to get a first feeling,” says Quanz, “and the answer was just overwhelming.” He recalls communicating his early calculations to veteran researchers more senior than him over dinner in around 2017. Guess how many exoplanets DARWIN could have unearthed, he asked them. One professor randomly hedged the number twelve, Quanz remembers. Stunning his listeners, Quanz told them that in less than a year, a mission like LIFE could pin down more than 300.
“It was just this excitement, knowing what this mission could deliver,” says Quanz. In the years since TPFI and Darwin, technology has progressed to the point where space-based nulling interferometry is no longer unthinkable. Instead, an initiative like LIFE is well on the way to being feasible. “I was just fixated by this idea,” adds Quanz.
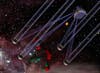
Flying space telescopes, reimagined
If nulling interferometry ends up being the next big wrinkle in space exploration, it will be because of young determined minds like Quanz, says Bertrand Mennesson, an astrophysicist at NASA. Mennesson himself was one of the scientists working on TPFI before he was diverted towards other projects. “It’s good to have new people looking at this and maybe coming to new conclusions,” he adds. One such example is whether the technology is reasonably achievable in the first place. The next step will require bringing together different space teams to put together a working prototype and vie for substantial funding.
High up on the to-do list is the demonstration of formation flying among autonomous spacecraft. LIFE will be made up of four separate collector spacecraft that gather infrared signals from exoplanets backlit against their stars, before redirecting the radiation to a central probe. For LIFE to work, scientists need to ready their propulsion systems, inter-spacecraft communication, and ability to hold steady to the precision of the wavelengths that they operate at. These autonomous infrared collectors will be spaced out thousands of feet apart, the deviation from their target positions can only be at most a tenth of the width of human hair.
The rise of small satellites (smallsats) and swarm technologies in recent years will be critical for whipping formation flying into shape to give LIFE—or nulling interferometry—a new lease on life. Smallsats and Cubesats in low Earth orbit are suitable prototype platforms to debut formation flying. A handful of orbital formation flyers have been deployed close to Earth—with several more slated for the near future—as testbeds to iron out various aspects of the technology. These incremental steps will prove the feasibility of formation flying in the decades to come.
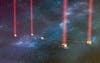
Another challenge for LIFE is to whittle the current landscape of infrared technology into shape. Fortunately, the development of JWST accelerated mid infrared optics that the new project will eventually make use of. Infrared is the preferred wavelength of light for planet-seeking interferometers, as many chemicals in planetary atmospheres absorb at this wavelength. Not only will LIFE have higher sensitivity to reveal new habitable planets in the first place, it may also deliver on its namesake—to allow scientists to study potential biosignatures such as methane and carbon dioxide on the planetary surfaces in further detail.
In the meantime, there’s no lack of effort to develop and make good use of Earth-bound infrared interferometers while scientists wait for one in the sky. This solution gets around formation flying, since individual telescopes can be shuffled more easily on the ground. (At Chile’s Very Large Telescope Interferometer, each of its four constituent auxiliary telescopes can be shuttled around atop trucks.) However, these telescopes have to contend with the nemesis of infrared astronomy: the atmosphere, which sponges up this radiation from the heavens. Turbulence in the air also blurs out these faint extraterrestrial readings—it’s like looking up and watching the outside world from the bottom of a swimming pool, says Barnaby Norris, an astrophysicist from the University of Sydney, Australia.
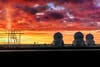
A new hope for the mission
As much as space-based nulling interferometry seems inevitable, space agencies have not drawn out concrete plans to carry it through so far.
Nulling interferometry isn’t one of ESA’s confirmed missions, and neither has ESA allocated funding specifically for a concerted commitment to develop the technology. “For the moment, it is not on our immediate agenda,” at least not until 2050, says Günther Hasinger, ESA’s director of science. “To put that technology in space right now is just too demanding.”
Across the Atlantic Ocean, NASA is actively pursuing alternative starlight-blocking technologies that are practically simpler but more suited to slightly shorter wavelengths. Mennesson says that these techniques aren’t necessarily substitutes for nulling interferometry, but can complement the latter in the search for extraterrestrial life.
[Related: With the arrival of Africa’s next radio telescope, Namibia sees a new dawn in astronomy]
Still, nulling interferometry’s ability to resolve distant Earth-like planets by honing in on mid infrared wavelengths is a niche no other exoplanet-questing method can fill.
“There’s obviously a lot of work to be done on the technology,” says Norris, “but I don’t think there’s anything insurmountable.”
Quanz says he will explore all avenues, such as approval from space agencies or collaboration with private entities, to bring LIFE to life.
He’s inspired by the very person whose mission LIFE has to thank: William Borucki, a retired space scientist at NASA Ames Research Center, California, and the principal investigator of the Kepler mission. It may be hard to imagine that Kepler, the most revolutionary exoplanet hunter humanity has brandished so far, had itself a rocky start: The mission concept was rejected by NASA four times since the early 1990s before it was eventually deployed nearly two decades later.
Quanz remembers getting this advice from Borucki at a conference in Cascais, Portugal in 2014: “If you’re really convinced by something … you have to stand up for it and just make it happen.” Following this star-studded legacy, LIFE will pick up where its predecessors left off and shoot for the skies.