

How will the universe end? Will it sputter out in a realm of ice, cooling continually as it expands until it reaches the absolute zero of temperature throughout its vast expanse? Will it die in a fiery blast as its component parts rush together faster and faster until they all meet in an enormous fireball? Or will the cosmos live on forever, expanding and contracting in relentless succession?
It is the ultimate question that man can ask, and it carries striking overtones of theology and philosophy. Yet, incredibly, astronomers think they will soon know the answer.
Their confidence stems from their success in answering, through a combination of observations and theoretical advances, a host of related questions over the past two decades. Did the universe begin in a spectacular explosion (the “big bang” theory) or was it simply always there, constantly renewing itself (the steady-state theory)? How did matter collect into the giant assemblages of stars we call galaxies? What is the nature of such bizarre entities as quasars and black holes? And what evidence do they contribute toward solving the ultimate puzzle?
The search for the answers to such questions has produced a picture of the universe fundamentally different from the one that existed before. Where cosmologists—the astronomers who make a specialty of understanding the nature of the universe—once thought that it was unchanging and serene, they now see it as dynamic, violent, and wracked by explosions on a scale too vast for human minds to comprehend.
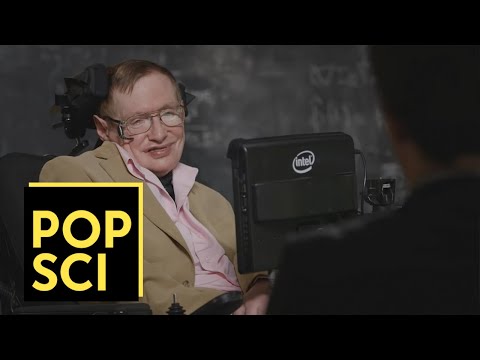
Strange, almost incredible objects populate the cosmos of today: cosmic vacuum cleaners named black holes, so dense that even beams of light cannot escape from them; tiny beacons of energy called quasars, no larger than the solar system, that emit more energy than millions of stars; and galaxies so vast that it would take an intrepid space traveler literally millions of years to cross them. Just as amazing as these objects is the fact that cosmologists think they can understand what roles the objects play in the life of the universe. “Rarely in the history of science has there been an equivalent period in which the boundaries of our understanding have been enlarged so dramatically,” declares Vera Rubin of the Carnegie Institution of Washington.
Cosmologists have argued about the development of the universe since 1930. In that year, a young astronomer named Edwin Hubble of Hale Observatories published a startling paper. Hubble had been studying the spectra of galaxies—the huge clusters of stars that populate the universe, most of them incredibly distant from Earth.
Spectral anaylsis of stars, galaxies, and other objects produces charts that show the exact frequencies of light and other radiation emitted by individual targets of astronomers’ curiosity. Scientists use the spectra in two ways. First, they obtain accurate information about the chemicals that exist inside a particular star or galaxy. Just as important, the experts can use the data to discover how fast the objects are moving relative to Earth.
Measurements of speed rely on the Doppler effect, named for Austrian physicist Christian Doppler who discovered it in the 1840s. It results when relative motion produces an apparent shift in frequency. The Doppler shift affects light, radio waves, and other types of radiation emitted by the stars or galaxies in the general direction of Earth. If a galaxy is approaching Earth, its light appears bluer than normal. If it is receding, the light looks redder than normal in a telescope.
When Hubble analyzed the pattern of red shifts, he discovered an astonishing thing: The galaxies, without exception, show a red shift—meaning that they are traveling away from us. Further, the more distant they are, the faster they are receding. The picture adds up irrevocably to a cosmos that is expanding. The expanding universe has almost become a cliche today, but it amazed astronomers of previous years who had learned to accept the universe as static and immovable.
How it all began
Careful studies more than a half-century have convinced all but a few maverick astronomers that the Doppler effect holds the key to the nature of the universe. But the results could be interpreted in two different ways. The arguments were published within a few months of each other in 1948. One, advanced by Britons Fred Hoyle, Thomas Gold, and Hermann Bondi, posited that the universe is infinite, without beginning or end. It expands, they argued, because matter is continually being created within the galaxies. American astronomers George Gamow and Ralph Alpher took a different view. They contended that the universe started with a big bang—a fireball that exploded in the beginning and flung all the pieces of the universe outward with a force that has kept the cosmos expanding ever since. Because of the enormous distances and times involved—the big bang certainly happened billions of years ago, according to the calculations of Gamow and Alpher—few astronomers had high hopes of deciding among the two theories. Then, in 1965, came a find that revolutionized cosmology.
It started with embarrassment for radio astronomers Arno Penzias and Robert Wilson of Bell Telephone Laboratories in Holmdel, New Jersey. They couldn’t get rid of a faint hiss in a radio antenna that they planned to use to study stars. The two men cleared a couple of nesting pigeons out of the instrument, scrubbed it thoroughly, took it apart and reassembled it, but to no avail. The faint interference continued, coming in at exactly the same volume from every direction in which they pointed the antenna. “It was,” said Penzias, “like cigar smoke in a room with no cigar.”
Then a chance meeting aboard an airplane steered the two astronomers to Princeton theorist P. J. E. Peebles, who had been studying the theory of the big bang. Radiation produced by the bang wouldn’t just disappear, Peebles reasoned. It would continue to permeate the universe, weakening in intensity but still remaining detectable eons after the big bang. It was that radiation, now known as the cosmic microwave background, that Bell Labs’ apparently balky antenna had detected. Two years ago, Penzias and Wilson won the Nobel Prize in physics for their discovery. To celebrate, they coined a twist on the old T.S. Eliot line: “This is the way the world began, not with a whimper but a bang.”
While the apparent triumph of the big bang over the steady-state theory now seems clear, there are still a lot of unanswered questions. For example, will the universe continue to expand and cool forever until it is dark, desolate, and frigid? Or will mutual gravitational attraction cause the galaxies to fall back together, only to explode again in a new cycle similar to the one we are now a part of? The prospects of a universe that expands forever, of one that contracts back and ends in a mighty reversal of the big bang, and of a cyclic universe bouncing back and forth might all seem equally unappetizing. Certainly no one has to worry about which will happen; the contraction, if it ever occurs, will not start for about a hundred billion years. But the issue is vital to astronomers because the fate of the universe is intertwined with its very nature.
The shape that space is in
In the universe, geometry is destiny. Cosmologists recognize that the universe can exist in one of two possible shapes. One can be pictured as the three-dimensional equivalent of the surface of a balloon that is being blown up. The surface has no recognizable edges or center, but it does have limited dimensions. A space traveler embarking on a long-distance trip in such a “closed” universe would eventually return home after circumnavigating the cosmos. He wouldn’t do so in the other type—the “open” universe. An open universe is shaped rather like a saddle that stretches away to infinity on all sides; the traveler would never return because such a cosmos is truly infinite. Mathematics tells cosmologists that an open universe will expand forever, while a closed one will eventually contract. Thus, by figuring out the eventual fate of the cosmos, experts can, in a roundabout way, determine its shape.
There are three different ways to tell whether the universe is open or closed. One involves measuring its total mass. If there is enough mass, natural gravitational attraction will eventually pull the galaxies back together. If not, expansion will go on forever. A second method is indirect. By measuring how much of the heavy form of hydrogen known as deuterium was produced in the young universe, astronomers expect to calculate its density at that time—and the power of its gravitational pull ever since. A third method requires measuring the rate at which the expansion is slowing down, by measuring the speeds of nearby galaxies and comparing them with the speeds of more remote objects that were embarked on their journeys early in the life of the universe.
The first method—measuring the mass of the universe- seems at first glance to resemble one of the labors of Hercules. Certainly it is complex, but astronomers can follow a few rules that simplify the task slightly. The key is the force of gravity. By observing the effect that enormous objects such as galaxies and clusters of galaxies have on each other through their gravitational attraction, astronomers can estimate their masses. Measurements of galaxies and galactic clusters suggest that the universe does not have nearly enough mass to be closed; it seems to contain only about one-third the required amount. However, a recent discovery could alter that picture considerably.
Earlier this year Dr. Frederick Reines, heading a team of physicists from the University of California, Irvine, announced a startling and still controversial find about the tiny subatomic particles known as neutrinos. Physicists had generally assumed that these elusive particles, theorized in 1932 but not actually identified until 1956, possess no mass. But the Irvine team, working at a nuclear reactor in South Carolina, decided otherwise. Neutrinos do, after all, have a tiny mass, they announced.
If their conclusion holds up, it could tip the balance between an ever-expanding universe and one that will eventually contract. According to current atomic theory, each thimbleful of space in the cosmos contains about 100 neutrinos. The mass of all the neutrinos in the universe could more than double the total mass, and perhaps provide enough gravitational pull to cause an eventual contraction. If that happens, Reines thinks that the universe will bounce back and forth between contraction and expansion. “There was no beginning and will be no end,” he prophesied when he announced his results at a physics meeting. “The consequences are theological.” The deuterium technique also yields uncertain evidence. But measurements to date of the amount of the isotope in the heavens, combined with current nuclear theory, point emphatically toward an open, infinite universe. Cosmologists caution that their science is hardly an exact one, that the conventional wisdom can easily be wrong—and that new discoveries can alter the picture entirely. “There is little doubt that other major components within our universe remain unknown to us today,” warns Vera Rubin.
One of the most important clues to the nature and destiny of the universe came in 1960. While Allen Sandage of Hale Observatories was using the Mount Palomar telescope, he discovered a series of strange-looking points of light. Astronomers called them quasars—for “quasi-stellar objects”—but nobody had any idea what they were until three years later. Sandage’s colleague, Maarten Schmidt, was looking at the spectrum of a quasar when he had a flash of inspiration. The extraordinary spectrum could be explained if it came from an object so violently red-shifted that the object was more than one billion light-years away. That find gave cosmologists their first hint that they could look back extraordinarily far into the history of the universe and hope to see something there. “Quasars fascinate me,” Schmidt declares. “It’s amazing that the universe manages to make itself known to us over such distances.”
For years now, Sandage, a cosmologist so reclusive that he recently had the telephone taken out of his office at Hale Observatories, has been making careful measurements in the attempt to see whether the differing velocities of the galaxies relatively near Earth (thus seen as they were in the relatively recent past) and the quasars (so distant that they are seen as they were near the beginning of the universe) give any hint to the cosmos’ ultimate fate. To date, Sandage’s calculations, based on relatively close galaxies, give a figure suggesting that gravitational attraction will never quite catch up with the outward force that the universe acquired from the big bang, and that expansion will go on forever—in other words, an open universe.
Sandage’s conservative view has recently been challenged by a series of experimenters who think the figures point more dramatically to an open universe. Researchers at the University of Texas and a combined team from the University of Arizona, Kitt Peak National Observatory, and Harvard-Smithsonian Center for Astrophysics have looked at some distant galaxies, and concluded that Sandage’s measurements are not typical of the universe as a whole. The reason: Our own galaxy is being pulled so rapidly toward the center of a supercluster that its movement relative to the rest of the sky makes all measurements in our galactic neighborhood unreliable. The controversy affects the past as well as the future. Sandage’s figures indicate that the big bang happened about 20 billion years ago. But if the new measurements are correct, the universe is only 10 billion years old.
As often happens in science, progress in one area spawns problems in another. The discovery almost two decades ago of the immense distances of quasars left astronomers with an embarrassing question for which an answer may be just now appearing.
The problem revolves around the fact that the light from most galaxies is not powerful enough to be spotted at distances of more than a few hundred million light-years. Quasars are many times that distance. Therefore they must possess sources of energy far more powerful than that responsible for starlight. The sources must also be extremely compressed. Meticulous observations of hundreds of quasars show that the mysterious objects are apparently no larger than our solar system. Until recently, physicists have been able to offer no explanations—even theoretical—as to how such an incredibly large amount of energy could be generated in such a relatively limited volume of space.
But now, the theorists have a strong candidate: the black hole. Many are now convinced that a black hole lurks deep inside each quasar, providing those objects with their penetrating power. In a way, black holes are as mysterious as quasars. They don’t seem to obey the normal rules of physics; they are, for instance, inherently invisible. Yet most astronomers accept that they exist, even though no one has found fully convincing evidence for one yet. “It is fascinating that black holes can be so bizarre and yet probably real,” says Kip Thorne of the California Institute of Technology, a foremost black-hole expert.
Hydrogen bows to gravity
As theorists first presented it, a black hole represents the death throes of a large star weighing perhaps three times as much as our sun. Stars usually obtain their energy from nuclear fusion, the conversion of atomic nuclei of hydrogen into nuclei of helium (fusion powers the awesome hydrogen bomb). The hydrogen lasts for billions of years in most stars, but eventually it runs out. At that point, gravity takes over. The monstrously large star starts to compress under the pull of its own gravity, becoming denser and denser and smaller and smaller. Eventually, the star might end up no larger than Manhattan Island, but with a density billions of times that of lead. It has become, in effect, a ball of immense gravitational force. Its gravitational pull is so powerful that no dust, gas, or even radiation nearby can avoid being pulled into the black hole. The force is powerful enough to keep anything from leaving the black hole—including light. In other words, it is theoretically, as well as physically, impossible to see a black hole. The only way to do it would be to approach so closely that one is swallowed up by the hole, disappearing into its core before one even has the chance to write down what it looked like.
Scientists wouldn’t be scientists if they rejected such a task as impossible. Astronomers admit that they cannot see black holes directly, but they think they can spot indirect evidence of them. The principle is to watch for material that is being swallowed by a black hole. According to theory, the material should give off particular types of X-rays as it disappears from view. If astronomers can spot those X-rays, they can say with some confidence that they have detected a black hole.
No one has yet claimed for certain to have achieved that, but astronomers using X-ray telescopes have found fairly firm evidence of four regions of X-ray emissions that appear to indicate black holes. The most promising is a region in the constellation Cygnus, the Swan, that was first spotted as a candidate for black-hole status in 1967 by Riccardo Giacconi, an X-ray astronomer now at the Harvard-Smithsonian Center for Astrophysics. More precise measurements of the X-rays should eventually prove whether the source, known as Cygnus X-l, really represents a black hole.
Quasar experts certainly hope that the proof comes soon. By understanding more about black holes, the experts think that they will be able to learn more about the mystery of quasars. It now also turns out that a better understanding of black holes may lead to the answer to another perplexing question that has been bothering astronomers. When the big bang happened, presumably there was a smooth, uniform mix of matter and radiation at high temperatures. How did it ever become the lumpy aggregation of planets, stars, and galaxies we see today? Astrophysicists studying this question have used the background radiation detected by Penzias and Wilson to make some astonishing discoveries about what the universe must have been like right back to its very first seconds. In fact, careful studies of the microwave background at various wavelengths have given cosmologists a plethora of information about the earliest happenings in creation.
To begin with, they have established that the big bang was very different from a conventional explosion, which sends material out from a single center. The event that started the universe happened everywhere at the same time—a mighty, uniform fireball that caused everything in the cosmos to expand at precisely the same rate in all directions. It is because of the immense power of that conflagration that the universe continues to expand today. But as it has grown larger, the cosmos has also cooled down, rapidly at first, then increasingly slowly.
Physicists cannot even imagine what conditions were like in the first few millionths of a second after the big bang. The cosmos was so dense and hot that current theories cannot deal with it. Amazingly, though, a combination of established theories and precise observations allows the experts to plot the subsequent history of the universe with a good deal of confidence.
About one-tenth of a second after the big bang, the density of the cosmos was about four billion times that of water, and the temperature had fallen to about one hundred billion degrees Celsius. That’s hot by most standards—far hotter than the interiors of stars, and much too hot for ordinary atoms and molecules to survive—but it is still cool enough for theoretical physicists to understand. Guided by experiments in particle accelerators, cosmologists speculate that the still expanding universe consisted of radiation—such as light—and elementary particles of matter, notably electrons, neutrinos, and positrons, which are identical to electrons except for the fact that they carry a positive charge. In addition, a few protons and neutrons, the building blocks of atomic nuclei, had started to emerge in the cosmic goo.
About three minutes later, the universe had become a little more comfortable. It had cooled to one billion degrees, low enough to permit some protons and neutrons to combine to form atomic nuclei of hydrogen and helium. In fact, the ratio of those two elements that was established three minutes after the big bang—73 percent hydrogen and 27 percent helium—foreshadowed the atomic constitution of all eras in the universe to come.
Matter and radiation continued to intermingle for a few hundred thousand years. During that time, the cosmos cooled to about 3000 degrees, the temperature at which the nuclei of hydrogen and helium could pick up electrons and form into conventional atoms. As free electronics disappeared in this way, matter and radiation separated from each other. The radiation became the microwave background, while the atoms of hydrogen and helium provided the raw material for the stars and galaxies.
Finding the center
How did the lumpy galaxies form from this homogeneous mixture? Most theories rely on some kind of small nucleus that attracted large amounts of hydrogen and helium to it, just as a speck of dust in a glassful of water cooled to its freezing point acts as the nucleus for ice to form. And one striking candidate for the nucleus emerges from the work of a young astrophysicist named Stephen Hawking. Hawking’s career is almost as fascinating as the subject of his research. Just 38 years old, he suffers from amyotrophic lateral sclerosis (better known as Lou Gehrig’s disease). He moves in a wheelchair, cannot write, can only talk in a mumble, and needs help to feed himself. But what Hawking can do, probably better than anyone since Einstein, is think. He composes long trains of complex mathematical equations in his head, and then dictates them, very slowly, to a specially trained assistant.
Since 1971, Hawking’s thoughts have focused on black holes. In particular he has pursued the idea that early in the universe, a great many miniature black holes, no larger than the diameter of a subatomic particle, were formed. These, perhaps, were the nuclei about which the galaxies coalesced.
Is there any way to test this revolutionary theory? Perhaps. As Hawking probed more deeply, he came up with an astonishing result: Black holes are not entirely black; they actually leak a little. At the time, Hawking admits, he was “rather annoyed” by the find. It seemed impossible. But further calculations in his head convinced him that he was right. What happens, he explains, is that the enormous energy in the neighborhood of a black hole can create pairs of particles spontaneously out of empty space. One of them, Hawking calculated, is likely to fall into the black hole, but the other may be able to fly away, giving the illusion that is has escaped from the black hole itself. Eventually, the entire mass of the black hole could dwindle away in this way, ending up with a spectacular explosion. The smallest black holes, Hawking calculates, might even be exploding about now.
The evolution of galaxies and their scheme in the design of the cosmos present yet other puzzles. Why are all known quasars at incredible distances—thus far back in the history of the universe? Many astronomers now suspect that quasars are actually galaxies in the process of formation. Perhaps they had their day of dominance at an early stage of the universe, and then largely disappeared. The disappearance could be explained if quasars changed into conventional galaxies soon after they were created. So far this picture is just a hunch, but new observations of more distant galaxies may settle it.
New devices may be the breakthrough
The observations accumulate, the theories proliferate, some mysteries even yield a bit. But the fundamental question as to the fate of the universe remains undecided, the evidence confusing. “One of the most amazing things is that the universe should be so close to the dividing line between collapsing and expanding,” says Stephen Hawking. But new instruments promise to settle the dispute. “We’re right on the brink of getting hard answers, and these instruments will push us over,” predicts Jerome Kristian of the California Institute of Technology.
Kristian refers to, among other things, tiny computer-controlled chips called charge-coupled devices, which astronomers are now attaching to their telescopes. They can detect spots of light with a precision previously undreamed of. Another approach is to make observations from outside the atmosphere. A 96-inch-diameter telescope to be launched aboard the Space Shuttle in 1984 will do precisely that.
Within the past decade, astronomers have exploited another powerful means of identifying stars and galaxies. Using high-altitude balloons, rockets, and unmanned orbiting satellites, the experts search the skies for the X-rays emitted by many objects in outer space. The picture that opens this article is an X-ray image of the remnant of a supernova (an exploding star), made by the “Einstein” orbiting astronomical observatory, HEAO-2 [PS, Feb.]. These X-ray observations yield new information on complex processes going on within such objects, and thus help astronomers to deduce new facts about their history and eventual fate.
Although the fundamental question remains unresolved, the evidence that does exist points—how strongly is debatable—toward an open universe. However, a number of astronomers remain unconvinced that the universe is open, on philosophical grounds. They have put their doubts in the form of what they call the anthropic principle. It states, simply, that the type of universe we can observe is a universe that allows human life to be created and survive. If the universe is open, it is presumably the only one that has ever existed. Given the chances against a cosmos that would be hospitable to man, our existence is at least as remarkable as winning at roulette with the same number 10 or more times in a row. On the other hand, if the universe is closed, it could perhaps keep bouncing back and forth in a cycle of different universes. If that is the case, the universe that we inhabit would be just one of many.
That type of philosophizing doesn’t appeal to many astronomers. They prefer to use their instruments to acquire new knowledge that will decide whether our cosmos is open or closed—and will add a multitude of other questions about creation. “We certainly do not understand cosmology, press reports notwithstanding,” says Philip Morrison of the Massachusetts Institute of Technology. Nevertheless, the fact that cosmologists can seriously try to solve the problem of the fate of the universe attests to their progress in recent years, and to exciting prospects for new achievements in years to come.
This article was originally published in the December 1980 issue of Popular Science.