What new insights about our first nuclear test reveal about the future of war
46 new papers continue to unfold the story (and power) of the Gadget
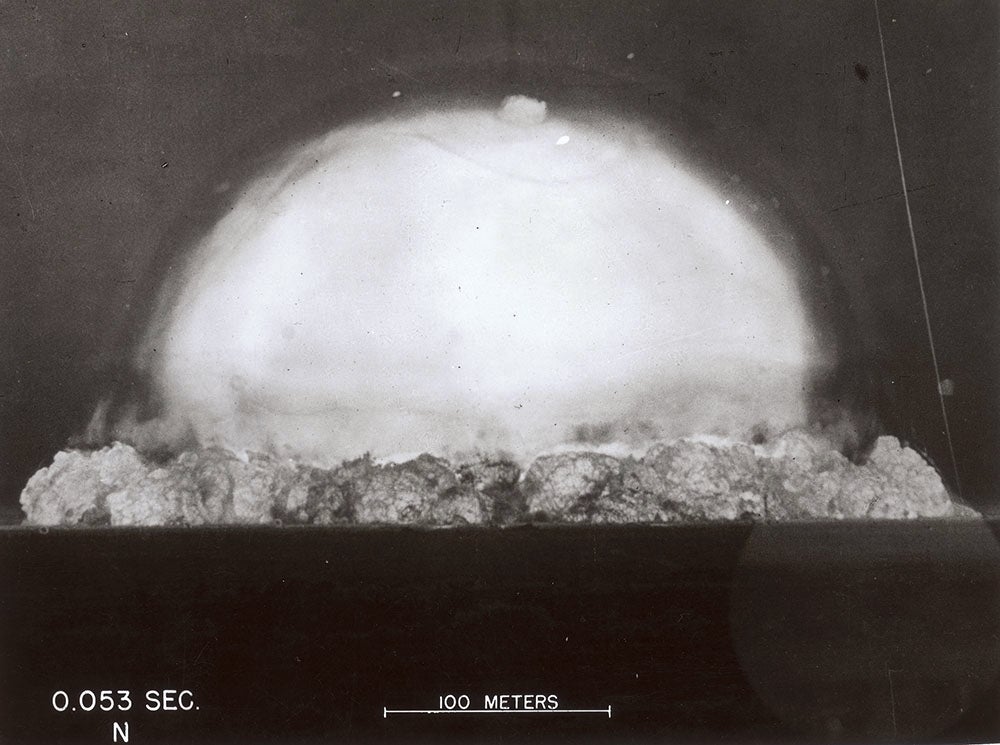
Films of the first atomic bomb detonation—a 1945 test called Trinity—are silent. Their quiet is unnatural, eerie. But in that hush, which you can not-hear on YouTube, it’s easy to imagine the sound that should assault your ears after the explosion’s flash, and the ensuing mushroom cloud’s expansion and rise. The cloud soon resembles a self-perpetuating storm, its edges turbulent, conflagrant, sucking up the planet beneath it as if for sustenance.
The scientists who’d made this bomb watched the Trinity test from a distance, welder’s goggles over their eyes and sunscreen on some cheeks. As part of the Manhattan Project, they had developed and built the so-called Gadget in just a couple calendar swaps, from a secret base in the New Mexico Mountains—a location named Los Alamos, which marks its 79th birthday this April, when the University of California signed a contract to operate the laboratory.
The modern Los Alamos National Laboratory was once part of a closed city. Today, a replica of the main gate is a public restroom and popular selfie spot. The city and the lab, though, pride themselves on their first raison d’etre: to figure out how to make a deadly device—and then do it, stat.
So when the 75th anniversary of the Trinity test came around in 2020, Los Alamos of course planned to mark the occasion.
Given what we all know of 2020, the typical sorts of commemorative events didn’t exactly come through. But one physicist, named Mark Chadwick, had a slightly unconventional commemoration idea that happened to be perfect for a pandemic: He proposed virtual lectures, followed by journal articles, that would reexamine Trinity’s science through the lens of modern tools and computers, thus both preserving and squeezing more knowledge out of that test, and its consequences. Understanding Trinity, and other past weapons tests, better helps scientists gather information about the state of current nuclear stockpiles.
The 46 resultant papers—covering everything from subatomic particles’ behavior to plutonium’s evolution to bombs’ yield to cancer risk—initially appeared in the internal Los Alamos journal Weapons Review Letters, a classified zine of sorts. And, after peer review and also security review, 23 papers went on to form a special issue of the journal Nuclear Technology, dedicated to the reexamination of Trinity.
The scientific focus of Chadwick’s lecture-paper project appealed to lab researchers like Susan Hanson, an inorganic chemist. “There’s a bunch of history of the Manhattan Project written by historians already,” she says. Not so much history written by scientists.
Los Alamos National Laboratory historian Alan Carr has heard a lot of quips and insights about that experimental day that took place almost 77 years ago. But his favorite comes from physicist Victor Weisskopf, because—like a weapon’s radiation—it spoke to a spectrum. “Our first feeling was one of elation,” said Weisskopf. “Then we realized we were tired, and then we were worried.”
The Gadget worked (elation :)); the scientists had worked hard on it (tired (-,-)); and they could sense how it might change future conflicts (worried (✖╭╮✖)).
Trinity, after all, resulted in devastating drops on Japan and ushered in the atomic age, the Cold War, a couple thousand more nuclear tests, the supercomputed simulations and experiments that eventually replaced those tests, and the current world order that relies in large part on who has nuclear weapons and who doesn’t. If knowledge is power, knowledge of this sort of power is maybe even more dynamite.
Chadwick, chief scientist and chief operating officer of weapons physics at Los Alamos, had long run a seminar series for his colleagues. But that became a problem around the same time the Trinity anniversary did: Typical talks contained classified information, which you can’t Zoom about from your home office in your pandemic soft pants (unless you’re into being arrested). And so, Chadwick thought, why not solve both problems at once? He could ask his peers to look at what photons modern science could shed on declassified aspects of Trinity’s near-century-old results. They could give lectures, and then (after a bit of conventional arm-twisting) write up their findings—an extra effort many agreed to, some simply because they couldn’t do their usual classified stuff from the kitchen table but needed to fill work-from-home hours.
Not being a hypocrite, Chadwick wrote one, focusing on how (and how well) his predecessors had done critical mass-related calculations: working out how much nuclear material they needed to create a weaponizable chain reaction. That question-mark was especially difficult to turn into a period, given that Manhattan-Project scientists had only tiny amounts of uranium and plutonium. “The country was working like crazy to produce enough material to actually make bombs,” says Chadwick. After a year or two of work, Chadwick found, the scientists were able to get their math within 10 percent of what we consider correct today—and he was able to add their original analysis to a modern database called Experimental Nuclear Reaction Data.
Inorganic chemist Hanson agreed to do back-research on Trinity’s radiochemistry, detailing how scientists first developed and then deployed radiochemical methods to calculate the Gadget’s “efficiency”—how good it was at making an atomic bang, and so how big that bang was. The device’s actual yield has always been uncertain.
The original radiochemistry team needed to collect samples of material in the crater Trinity left behind. From them, they could figure out what portion of the plutonium had split and how many fission products there were. “You couldn’t just walk in there and pick them up with your hands,” Hanson says. At least not if you liked your hands, or your life.
Instead, the researchers lined two tanks with lead. One, with a trapdoor underneath, drove straight into the crater, and sucked samples from its center. The intrepid, air-filtration-wearing explorers inside this extra-armored vehicle were the first to see “trinitite”—the glassish substance that formed when the hot, hot heat of the atomic blast met the desert sand. To the second leaden tank, scientists attached rockets, their noses equipped with scoops, their tails attached to cables. After the rockets passed over to the crater, workers could wind them back into the tank as if they were fishing lures.

These samples, and the ratio of their plutonium to fission products, led scientists to calculate the Gadget’s yield to be 18 kilotons—the equivalent of 18,000 metric tons of TNT. Later, that figure was revised upward to 21 kilotons.
As part of the anniversary project, Hanson and colleagues worked to re-recalculate that yield, by dissolving 13 original samples of trinitite. New methods—a combination of nuanced techniques like inorganic separations chemistry and ultra-precise mass spectrometry—can pull out an element of interest, and then more precisely measure the isotopes within. Computer-aided analysis of those ratios gave a new quantification: 24.8 kilotons.
Trinity, in other words, has gotten more powerful with time.
Much of the work on these papers wouldn’t have been possible without a strange place called the National Security Research Center. “Think of Raiders of the Lost Ark,” says Chadwick. It’s a library of Los Alamos archives, many not available for public consumption, dealing with the lab’s history—which is in a lot of ways the history of nuclear weapons themselves. The library, one of the larger libraries of any kind, houses around 20,000 documents about the Los Alamos branch of the Manhattan Project, called Project Y. They live here in carbon and, when someone has taken the time to scan them, silicon format.
Even Carr, the lab’s historian, hasn’t explored it all. In fact, his Trinity-anniversary work—on a paper called “Thirty Minutes Before Dawn”—led him to a banker’s box of records he’d never seen. Field notebooks contained the original information about potential health hazards from Trinity: pages of the pencil-scrawled radiation readings from workers who trekked to areas near the explosion site and took Geiger measurements. “As I’m going through records, I’m like, ‘Wow, these were in the fallout produced by Trinity,’” says Carr.
History was literally washing—or zipping—over him. Maybe, he thought, he should have someone come Geiger-count these papers, before he started poring over them.
“There’s always been a debate about this: Are nuclear weapons our damnation, or are they our savior? A lot of this is unknowable.”
Alan Carr, Los Alamos National Laboratory historian
It’s mostly a joke. That particular history isn’t really dangerous to him now, time having rendered it inert enough. But it’s also true that scientists can only estimate how radiation from weapons tests like Trinity affected people’s health and the environment, in part because of the limited data that exists.
The radiation from that earliest test does, after all, still persist—not just in archived papers but also in the parts of the planet close to the test. At the site of the Trinity test, you’d get half a “millirem” of radiation in an hour, which, if you stood there doing nothing else for a year, would be about seven times the average dose from all natural and human-made sources. That lingering is, in fact, part of the point of the first and subsequent gadgets. Radiation doesn’t just go away.
And neither do nuclear weapons in the American arsenal. That’s part of why, at a place like Los Alamos, looking to the past isn’t just about revisiting the (ethically complicated) glory days: It’s about evaluating the present and presaging the future.
After Trinity, the national labs conducted bomb tests on the regular, both above- and below-ground, gathering data on whether and how the weapons worked. “Those are things that we can’t do anymore,” says Carr, the US having signed (but not ratified), the Comprehensive Nuclear-Test-Ban Treaty.
It’s more difficult to tell if our existing nuclear stockpile is, as insiders are fond of saying, “safe and reliable” if you can’t just blow a few of its weapons up and see if they detonate when and how they’re supposed to, and don’t detonate at all when they’re not supposed to. That okay-or-nay assessment involves, instead of a cloud-anticipating countdown, smaller-scale experiments and computer simulations that together can help reveal the weapons’ internal states.
Understanding what all of that not-quite-a-test information means for a full-scale bomb is where the archives come in. “A lot of this relies on data that was created during the Cold War,” says Carr, during the full-scale testing era. That’s why Carr’s job is to know what was done in the past—so it can inform the present, and help new employees with their strange orientation. After all, says Carr, “they don’t teach you things like nuclear weapons design in college.”
“Fortunately,” he adds.
Designing the Gadget, and doing the Trinity test, were formidable technical achievements, taking a nascent branch of physics and turning it into a physical (and physically destructive) object in less time than it takes to add some lanes to an interstate. The Manhattan Project was arguably the first Big Science program, a meeting of famous physics minds high on Enchanted-State mesas, and involved the labor of more than 100,000 workers across the country. But making an atomic bomb wasn’t just a technical wow showcasing new science, innovative engineering, and the military-industrial-academic complex: It was a long-term, people-killing plan that would alter international relations forever. “You have to acknowledge that within a few weeks, these weapons were deployed over Imperial Japan,” says Carr. “That’s a loaded history.”
The motivation for having kept nuclear weapons around is somewhat different from the motivation for developing the Gadget in the first place. Trinity represented a proof of battlefield concept, and, in a lot of ways, a simple if-then query: Did the bomb work? If yes, then explode one or more above the heads of our enemies.
Today, meanwhile, nuclear weapons exist to act as a deterrent (or so the philosophy goes). We keep them so that they keep other countries from exploding their nuclear weapons in our general (or our allies’) direction. The strategy, though, only holds if the weapons will work when called upon, and if the other side believes they could credibly be called upon. “Their value as a deterrent relies on their ability to perform—without question—in combat,” says Carr.
As the Russian invasion of Ukraine, and the ongoing war, show, deterrence is not simple. “Deterrence is neither as stable as some strategists insist nor as easy to escape as some disarmament advocates hope,” Matthew Harries, a senior fellow at a UK think-tank called the Royal United Services Institute, wrote in Foreign Policy. “Wherever the war goes from here, it’s clear that nuclear deterrence is not automatic or inherent to the mere possession of nuclear weapons.”
It was Trinity that first ushered in that delicate, counterintuitive, contested balance in the first place. We have nuclear weapons to keep the peace; we have nuclear weapons because the Trinity test worked; the Trinity test only worked because we had a nuclear weapon.
“There’s always been a debate about this: Are nuclear weapons our damnation, or are they our savior?” says Carr. “A lot of this is unknowable.” That uncertainty holds regardless of how powerful your supercomputers or modeling methods are. Yet there’s even more greyness to it. For instance, the benefits of deterrence aren’t evenly distributed. In Ukraine, Russia’s nuclear-armed status has made NATO countries act more cautiously, Harries pointed out, which means more damage to the invaded country and its people. And the whole philosophy relies on the destructive threat being credible. “Because deterrence is neither automatic nor static, there is no way to reap its prime benefit—discouraging war between nuclear-armed states—without some real chance of nuclear weapons being used, even if the probability is low,” he continued.
That probability is now, and always has been, unknown—if not unknowable. So too, though, are the pure, precise details of how nuclear weapons work and how they age. The digital codes and models that scientists use to mimic bombs involve estimation (like most codes and models do), because we can neither write nor run software that truly replicates the physical weapon. These are very good estimations, but they nevertheless have assumptions and approximations embedded inside—about how atoms and materials and explosives behave in various configurations and environments, and are not exact reflections of reality. You can create something, in other words, and not fully understand it.
Correction 5/2/22: An earlier version of this article incorrectly stated that 18,000 kilotons of TNT is equivalent to 18,000 sticks of dynamite. It’s way more than that.