Researchers just linked three atomic clocks, and it could change the future of timekeeping
Redefining the second is a big project. This is one crucial step towards accomplishing that goal.
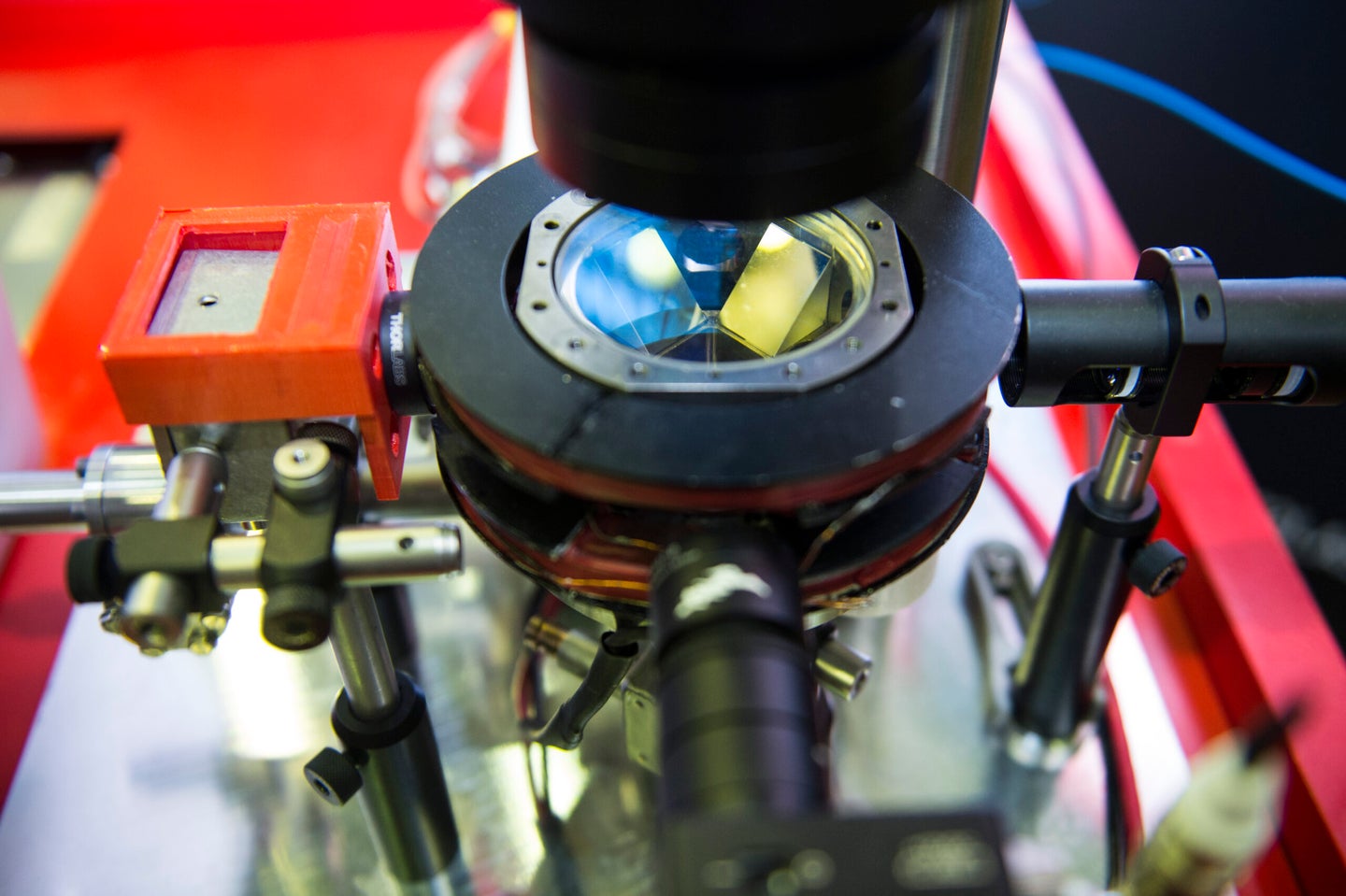
A towering pendulum clock loses or gains around a minute every month, while the quartz in a digital watch stays within a few seconds. But at the bleeding edge of timekeeping, scientists are fine-tuning clockwork that might stray no more a second over the course of billions of years.
It might seem trivial to bother improving a clock that’s already so accurate, but it’s crucial for a few areas of science. Better clocks could fine-tune researchers’ ability to measure relativity and dark matter. They also help geodesy, the science of measuring the Earth itself, allowing us to map its gravity, its surface, and its sea floor to within centimeters. And since systems like GPS rely on timing signals, they could unlock a new generation of navigation technology.
That’s partly because creating ever more accurate clocks enables scientists to better define the second, our base unit of time. While most of the standard SI units were redefined in 2019, the second wasn’t one of them. In fact, the second’s definition hasn’t changed since 1967.
But now, dozens of researchers in Boulder, Colorado, working together in the Boulder Atomic Clock Optical Network (BACON), have been able to connect three of the world’s most accurate clocks together in a critical step towards creating a new definition. By linking different clocks, researchers can check to make sure they’re all reliably keeping time.
And once that can happen, it paves the way for this newer, more accurate type of clock to redefine the second. BACON’s measurements, recently published in Nature, are around 100 times more accurate than even the ultra-accurate atomic clocks that the planet’s official timekeepers use today.
Today, we define the second with atoms—in most cases, cesium-133. In particular, we look at the frequency of the photons that are absorbed and emitted when electrons shift energy levels, which in a cesium-133 atom are microwaves. You can use the period (the time between the peaks of a wave) of those microwaves to keep time. To wit, exactly 9,192,631,770 of those periods make up one second.
That sounds impressive, and it is—but it’s Cold War-era tech. BACON and other projects are tinkering with a much newer type of atomic clocks, called optical clocks, which rely on light. Because light has higher frequencies than microwaves, and thus shorter periods, you can use that light to get much more accurate timing.
The latest cesium clocks are accurate to within a millionth of a billionth of a second. To show that optical clocks really are the future, scientists need them to be 100 times more accurate than their cesium-microwave predecessors—a benchmark they hit in this new study.
But they also need different timepieces around the world to agree on the time, especially because not all optical clocks use the same atoms. That isn’t easy. Not only do the clocks themselves operate differently, effects of relativity make themselves known. Atomic clocks at different altitudes tick at different rates, thanks to subtle time dilation caused by Earth’s gravity.
[Related: How the heck do you even add a leap second to a year?]
Boulder, Colorado is fertile ground for linking optical clocks because no fewer than three live there—two at the NIST laboratory and a third about 0.9 miles (1.5 kilometers) away at JILA on the University of Colorado, Boulder, campus. The three clocks all work with different atoms: Strontium-87 at JILA, and aluminum-27 ions and ytterbium-171 at NIST.
There was already one link between the two sites. “We’re lucky that there’s a fiber-optic cable that actually runs under the city of Boulder that connects the clock at JILA to the clocks at NIST,” says Holly Leopardi, a researcher at the University of Colorado, Boulder and NIST. It’s a well-established method of linking optical clocks at the accuracies scientists want; for instance, researchers have built a fiber-optic network connecting clocks across Europe.
“But you’re always worried that maybe there’s…some issue that has crept in that you’re not aware of, or something like that,” says David Hume, a researcher at NIST. “So we wanted, as much as possible, redundancy in this network.”
With that in mind, the researchers created a second link above ground. They constructed a system to shoot laser pulses between the top of a NIST building and the top of the 11-story Gamow Tower on the university campus.
BACON researchers then had two different ways of measuring the ratios between the frequencies of their clocks, allowing them to check that both the clocks and the links were reliable. Both numbers indeed fit within the ultra-accuracies they wanted.
“We had one day when we didn’t have those overlapping measurements, and that was because there was a snowstorm,” says Hume. “We couldn’t send the laser light through the snow and get enough signal on the other end.”
When you’re trying to time billions of billions of ticks each second, even utterly minute factors such as signal loss in the cables and turbulence in the atmosphere can throw off your pulses. The researchers had to repeatedly check that their links and their measuring systems weren’t adding error.
“The really cool thing about the free space system is that it’s able to measure all of the noise and turbulence in the atmosphere,” says Leopardi, “and we’re able to correct for it.”
Scientists eventually want to use this technology to create a global network that would, for instance, link optical clocks in the US to their counterparts in Europe or East Asia. We can’t do that just yet—there’s too much error over such long distances—but scientists hope to soon create, for instance, a satellite network that harnesses the work of BACON’s free-space link.
“I think it’s really amazing that we’re able to all collaborate and get together to actually perform this measurement and work together to get these results,” says Leopardi.