

From the next room, through a thick granite wall, comes a chug-a-chug-a-chug-a, like an old steam train closing in. Rounding the corner, I see the source of the racket: a table, shaking. The long, metal slab jerks quickly back and forth. On it, in two neat rows, are a half-dozen rectangular prisms packed with sensors measuring pressure and motion. Each one holds a titanium-alloy bolt the size of a grown man’s forearm and weighing about 10 pounds. As the elaborate assemblage might hint, these bolts are special.
Eventually, this remarkable hardware will go to space. The bolts, or ones like them, will hold together sections of the Orion spacecraft, a new vehicle that, sometime in the next decade, will carry humans out of low-Earth orbit for the first time since 1972—initially to the moon and later on trips to Mars. But before that, the fasteners must survive a mock version of their journey. Only worse.
The shaking they’re enduring is merely the beginning, intended to simulate the violence of a launch. The parts also brave hammering, baking, and freezing—24 tests in total. All this before any metal even reaches the launchpad. The abuse ensures not only that the bolts will hold together massive space-faring machines, but that, at the exact right moment, they’ll break neatly apart. More specifically, they’ll explode, strategically jettisoning segments of Orion’s rocketry as they do.
The design, manufacture, and most of the testing of this combustible hardware happens in an old stone factory in Eastern Connecticut, where engineers have crammed various items full of pyrotechnic material for well over a century. The 200-acre campus of 19th-century brownstone, granite, and brick—a look that’s part factory town, part college—is the home of the Ensign-Bickford Aerospace & Defense Company (or EBAD, because what’s a defense contractor without a vaguely sinister acronym?). EBAD is one of more than 2,000 companies making Orion’s nuts and bolts (and ceramics, fabrics, and springs) for Lockheed Martin, NASA’s main contractor on the project.
EBAD’s components are a bit player in this space epic, but the firm’s mission-critical role gives it an outsize gravitational pull. Of the 5.5 million pounds of rocketry (collectively known as NASA’s Space Launch System) and other equipment that will hurtle Orion out of the atmosphere, only 20,500—less than 0.38 percent—will come back to Earth. “The last thing we want to do is take all the stuff at launch to the moon and back,” explains Carolyn Overmyer, Lockheed’s deputy manager for the Orion crew capsule (where the astronauts ride). “We don’t need the blast system at the moon. So where does it go? It separates. It’s a ‘sep event.’” In plain English: Stuff falls off.
The exploding bolts are the catalyst in that process, “central to our mission,” Overmyer says.

Right: An industrial oven broils parts to test their mettle. Ray Lego
There are eight separations in a complete Orion journey to the moon and back. One of the first occurs three minutes after launch: The bolts split alongside explosive-powder-laced zippering fissures called frangible joints to discard the loads that get Orion off the ground. Three nearly-two-story panels, called fairings, that protected the craft from the heat of liftoff simply drop. “A 15-foot-tall coffee can goes boom! and just flies away,” Overmyer says, recalling the first time she watched the panels split from the craft during a test flight. “I know it sounds silly to say it, but I found it very, very beautiful.”
As the mission progresses, more systems become irrelevant and break off. The final thing to go is the service module, a trash-can-shaped pod that houses all the liquids and gases for the mission; it holds on to the Orion capsule throughout the 1.3-million-mile journey on the strength of four fasteners made for this exact task. When the crew-carrying vessel begins its dive back to Earth, the fasteners split and release the pod, which then burns up.
Preparing these bolts for their pivotal moment—their perfect failure—presents as a kind of Zen koan. How to fully test a thing that works exactly once? How do you design something that, in order to do its job, must fail?
Part of the answer is revealed in the threaded fasteners, called release and retention bolts, shaking and rattling on the table. Of all the variations of hardware EBAD builds for Orion, these must suffer the most intense torture, both here on Earth and in space. “We beat the hell out of ’em,” says Steve Thurston, EBAD’s manager of test services, as he watches the heroic fixtures rumble angrily against the table’s motion. Thurston turns and walks toward a quieter spot and says softly, almost solemnly: “It’s really not fair to the parts. But that’s the point—to find their limits, to push the envelope.”
Outside, a morning rain gives way to the bright-green beginning of a fall day. A river, which once powered EBAD’s works, winds through the campus; a family of otters has taken up residence. It’s hard to square the setting with what goes on behind the aged stone walls: space-age bolts getting stretched (and mashed and bashed and rattled) to their limits.

Simsbury, Connecticut, has been home to EBAD since well before the Civil War. Back then, there were iron and copper mines and granite quarries throughout the region, which meant a lot of digging and an awful lot of booms. The methods were crude: Dig a hole, fill it with gunpowder, plug it except for a small space to run a fuse (usually string or cloth), light, run. Men died by the hundreds, often because things blew when they weren’t supposed to—mostly too soon.
In 1831, these techniques began to change—become more refined, predictable, safe. In the county of Cornwall in Old England, where there was even more mining than in New England, an inventor named William Bickford patented the first safety fuse. Bickford packed gunpowder into a hollow jute rope, which then fizzled at a predictable clip of roughly 30 seconds per foot. In 1839, he partnered with a Connecticut mining company to manufacture and sell his burners stateside. Ralph Hart Ensign joined on in 1870. His heirs would later expand the firm’s explosives business beyond fuses, developing products such as a banker’s bag that smoked when a crook tampered with it.
Dave Novotney, head of business development, quickly walks me through this century-plus of history as he winds up to a crucial point. He pushes back from his desk and lays it out: “We blow things up here. We are very good at it. We’ve been doing it for a long, long time.” But the key, even in Bickford’s day, is timing. Timing was—and still is—everything.
For no one is this truer than the astronauts inside a small hunk of metal hurtling through space at 20,000 miles an hour. Which is why, almost paradoxically, in missions with humans, there’s explosive powder planted in dozens of spots throughout the craft. It does what you want it to do when you need it to.
NASA doesn’t call these propellants “explosives.” Instead, they’re pyrotechnical systems, or pyro, in which so-called separation bolts are a central part. An electronic switch called an actuator delivers a charge to a threaded incendiary cord that leads to the fastener. The event is over in a fraction of a millisecond—about one-millionth of a blink of a human eye.

The space agency has relied on this type of fast action, also common in ejector seats and weapons deployment, from its beginnings. The Mercury missions of the late 1950s and early 1960s experimented with pyro, though not always with stellar results; an escape-hatch misfire at splashdown during the Mercury 4 flight flooded the capsule and nearly drowned an astronaut. NASA got better at controlling booms by the Gemini program of the mid-’60s, which added new structures such as pyrotechnically deployed landing gear. By the Apollo missions of the late ’60s and early ’70s, 210 bits of explosive tech took on 24 mechanical functions—from separating the lunar lander to releasing parachutes for descent—on the crafts that first brought humans to the moon. EBAD provided pyro for many of these small-but-mighty ignitions.
The explosions, however, took a backseat during the shuttle program. The space planes relied more on motorized, reusable systems—ones that dock and undock attachments, including spacewalking astronauts. Motors, however, aren’t perfect, Stu McClung, a NASA engineer who works on Orion’s pyro and spent nearly two decades on the shuttle, later explains to me. They’re several seconds slower than pyro, can be pounds heavier, and worst, they can also break down. So he still favors detonations as a failsafe. “If something ever went wrong, we could just fracture it, and head on home.”
Today, electric action is increasingly in demand for satellites and unmanned systems, such as the James Webb telescope and the OSIRIS-REx asteroid sampler, which have solar arrays that need to gently unfurl. “The good news is pyro systems act really quick,” Novotney quips. “The bad news is they act really quick.” Also sad for our lug-headed friends: SpaceX founder Elon Musk, whose ethos centers on reusability, is not a fan of the bang.
EBAD had very little kit on the shuttle, and years ago, Novotney noticed most space business was moving away from pyro altogether. Orion, though, is a throwback to the pre-shuttle era—a time reliant on controlled detonation—and a chance for the company to leverage its explosive bona fides. So Novotney bid, eager to catch a once-in-a-generation craft before it flew by.
Now he and a team of engineers are somewhat obsessed with the trial and error of bolt-making. The end result of their work occasionally winds up in Novotney’s office, in a yellow bucket deep with shards of spent fasteners he likes to show off to visitors. Peering down at this refuse, it looks more like discarded lengths of filled-in pipe than hyperengineered and endlessly tested exploding space stuff. Their task is dead simple yet takes years to perfect: Stay together, break apart, help deliver the crew back to Earth. “It’s not like you can suddenly change your mind and send Bruce Willis out on a rescue mission,” Novotney says. “You’re coming home, and that’s it.”

EBAD has been alternately making and breaking Orion hardware since 2009. When it began, Lockheed delivered reams of documents with specs, first a few hundred pages, then several thousand more. Still, EBAD wasn’t starting from scratch. The company established a Space Ordnance Division to make specialized fuses in 1965, and a handful of other companies—even NASA—had been crafting separation bolts since the ’50s. So, the broad strokes were, by the early aughts, already out there.
To perfect a bolt that also must, when called upon, be decidedly unboltlike, EBAD engineers spend an outsize amount of time worrying the hardware’s weakest point: the fracture plane, the epicenter of the eventual break. Lockheed’s Overmyer likens it to folded paper: “When you bend it, you make the crease really strong so it breaks at that line,” she explains. If the hardware was going to split too soon, like during launch, it’d happen here. On a bolt, the plane is a razor-thin notch circumnavigating the titanium surface, about two-thirds from one end. In EBAD’s early testing days for Orion, the designers fiddled with the placement, the depth. Most important, they carefully paired it with the internal structures and explosives that would ensure a clean break at the right time, every time.
As they work on their refinements, the rules keep changing. Halfway through development—after more than a dozen bolt iterations and a couple of vehicle tests—NASA determined the Orion service module needed to go on a serious diet to make room for the systems that would support human life for its eventual two-year trip to the Red Planet and back. About 3,000 pounds had to disappear from the then-49,000-pound vehicle. For EBAD that meant fewer, burlier bolts. Instead of six fasteners that would need to last nearly the entire journey, they’d have four, which saves about 25 pounds. “This caused some headaches,” says Sean Keon, an EBAD engineer who oversees design. They made the bolt all over again, adding some girth and about a quarter-inch of length. The tweaks allowed each to hold more than 100,000 tons, so Orion could lose two without concern.
RELATED: What could life on Mars look like? This Chilean desert holds some clues.

The team machines the bolts according to exact specs; if their measurements are off by more than 1⁄1,000 inch, they’re no good. But the real trick to building these Herculean fasteners isn’t building them. It’s test after test after test. In addition to the shaker table, all the parts run through trials that simulate versions of the mission’s extremes. EBAD freezes them down to minus 100 degrees and heats them to 210 degrees, which ensures their fuses won’t spontaneously ignite midflight under the sun’s glare. To prove the bolts can hold tight through the shock wave of rocket ignition, they suffer three 6,000‑G whacks from Thor-worthy steel hammers.
Throughout the process, engineers check and recheck the bolts. They remeasure them to make sure their forms haven’t yielded under pressure. X-rays ensure that all of their internal parts are present—and in the right place—and a fluorescent dye highlights cracks as tiny as 0.03 inches long. Once EBAD is satisfied, some nine fasteners from each manufacturing batch head to Lockheed and NASA, which put the pieces through more abusive paces. If any of the hardware has a bad test or a fissure, EBAD pulls the whole lot, and the process starts all over again.
[Related: Sputnik-2 or: Laika, Our Hero]
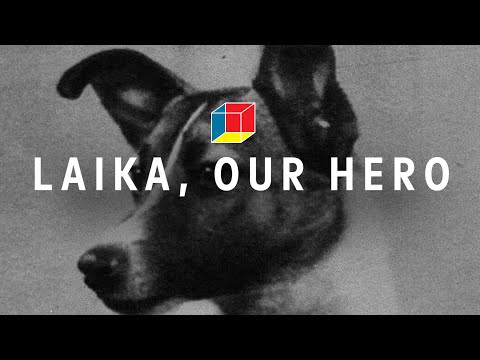
Another way to monitor the success of an explosive space bolt is to watch it blow. This happens so fast that observing the boom is terribly underwhelming. There’s almost nothing to see. Like magic. Only it’s not.
The only way to really “see” the explosions is in slow-motion video running at a fraction of the rate human eyes can naturally glimpse—at least 100,000 frames per second. And even then, plenty remains hidden, including the electrical charge that sets off a series of tiny detonations that ultimately ignites an organic propellant inside a pressure cartridge. The propellant generates enough energy to drive two internal pistons. The pair then slam against one another with enough force to cause the all-important fracture plane to finally, perfectly, once and for all, fail. From the outside, it looks like the bolt is pulling itself apart.
Spacecrafts have redundancies everywhere, including inside the bolts. There are two pressure cartridges, next to each other. If the primary one doesn’t fire, the electric charge continues on, tapping and igniting the second. If both fire at the same time, which sometimes happens, the shell can still contain the force.

But the job isn’t finished once you pull off the explosion. The break itself can cause problems, because, in space, debris is a killer. A tiny fragment of bolt, hurtling around Orion at thousands of miles per hour, could easily smash a solar panel or pierce an important piece of electronics, ending the mission. This is why, when the fasteners nestle among their testing sensors and ultimately snap in two, there is a little baggie dangling underneath to capture debris. Lockheed analyzes the refuse to ensure there are no pieces large enough to cause problems. They review the slo-mo tape too, checking the velocity of anything shooting away from the fracture and the craft.
Being certain about where broken parts wind up is doubly important for the most heroic bolts, the ones that secure the crew capsule to its trash-can-shaped life support until the mission’s near-final moments. Pieces of that hardware must stay on the craft, and contribute to another vital feature. After the bolts split, the shards remaining on the capsule melt slightly and become part of the heat shield, throwing off excess heat and helping protect the astronauts during the 4,000-degree push back into Earth’s atmosphere. As they melt, they take the heat with them—like chunks of ice sitting on blacktop on a hot day.
As Keon and a group of EBAD engineers describe these final throes, I catch them staring at the conference-room wall behind me. Tucked near the ceiling is a rolled-up projector screen. Test flights are the only real chance for the bolts to prove their mettle, so when they happen, EBAD staff huddle in this room to watch. Right now, NASA is inching toward two big events: a four-minute ride will practice an emergency landing this spring; and, in 2020, Exploration Mission 1 will whip an unmanned capsule around the moon and back home.
The last time they piled into this room was in 2014, when Exploration Flight Test 1 circled Orion around Earth twice before splashing down. The unnamed mission was a trial for critical systems such as the heat shield, parachutes, computers, and, of most concern for EBAD, all those separations. That mid-December afternoon, the team ordered pizza, and waited into the night to see how their bolts fared. They paced and sweated, then let out cheers and long-tired sighs. The celebration, though, was tempered by the work—the testing, the refining—they’d return to the next morning. “The mission’s not over,” Keon says.
This article was originally published in the Spring 2019 Transportation issue of Popular Science.
Correction 10/13/21: An earlier version of this story mis-identified the county of Cormwall in England as a city.