

A precisely arranged triangle of three satellites shooting laser beams at each other truly sounds like science fiction. But the European Space Agency (ESA) is set to make this a reality by 2035.
The project, known as the Laser Interferometer Space Antenna (LISA), is like the famous gravitational-wave-discovering LIGO experiment—just in space instead of underground tunnels. Lead by ESA, the project is a collaboration with NASA and a consortium of scientists. ESA recently gave the mission team the official go-ahead to begin construction on the spacecraft in January 2025, with launch planned a decade later. Astrophysicists who work on the mission, like Max Planck Institute astrophysicist Sarah Paczkowski, were overjoyed at the news, describing the mission adoption as “rewarding” and “super exciting.”
“LISA will be sensitive to an as-of-yet unexplored regime of gravitational waves” or ripples in the fabric of spacetime, explains Michael Zevin, an astrophysicist at the Adler Planetarium and part of the LIGO collaboration. Gravitational waves reveal the physics of black holes smashing together, massive supernova explosions, and even the earliest moments of the universe. LISA’s new perspective is “akin to the first time observing the universe in light outside the visible range, such as X-rays or infrared, which enabled an immense amount of science, discovery, and understanding of the cosmos,” he adds.
Since the first detection of gravitational waves in 2016, astronomers have been eagerly exploring the cosmos through this new window. We’ve heard the final moments when two black holes spiral into each other and learned about how the highest energy events in the cosmos—like supernovae and gamma ray bursts—happen by simultaneously spotting light and gravitational waves from a cosmic explosion.
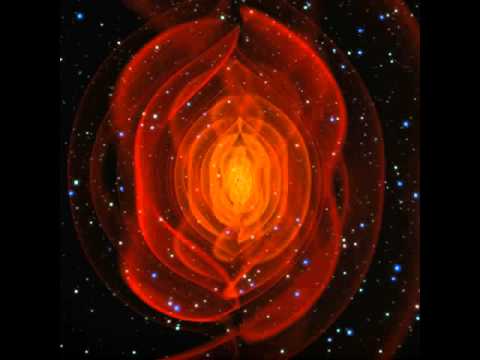
[ Related: Gravitational waves just showed us something even cooler than black holes ]
The common words between LISA and LIGO are laser interferometer, describing the setup of the experiment. These projects note how the distance between two objects changes ever so slightly as a gravitational wave ripples by. They do this with two long arms—in LIGO’s case, two big tunnels each 2.5 miles long. In those tunnels, there are huge vacuum chamber tubes where laser light travels down each arm and bounces back off of a mirror. Where the light recombines in the center, it’ll look different if the laser has to travel farther in one arm due to a passing gravitational wave.
But, Earth-bound experiments are limited in the type of gravitational waves they can detect. With gravitational waves, you need long tunnels like LIGO’s to notice the ripples—and the longer the arms, the longer the waves you can detect. Underground detectors like LIGO are best at spotting the shortest gravitational waves, very high frequency vibrations in spacetime that come from the last milliseconds before black holes or neutron stars collide. This is partly because Earth is absolutely buzzing with activity that can disrupt the detector. “Earthquakes, cars, ocean waves, and even clouds passing over the detector” create noise that prevents astronomers from hearing the low-frequency rumbles of the universe, says Simon Barke, LISA Charge Management Device scientist at the University of Florida’s Precision Space Systems Laboratory.
Ground-based detectors are limited in the size of the experiment; we simply can’t create a building bigger than Earth, or even bigger than a few states, limiting us to the shortest gravitational waves. On the other end of the spectrum, pulsar timing arrays like NANOGrav can only hear the lowest frequencies originating from humongous supermassive black holes, as they’re measuring the vast distances between dead stars known as pulsars. For everything in between, you need LISA.
“We’ve so far only heard a very narrow range of the sounds of spacetime,” explains Zevin. “Detectors on the ground have been listening to the violins of the gravitational-wave symphony. Pulsar timing arrays have recently announced evidence for hearing the bass section from supermassive black holes throughout the universe. LISA will be the first space-based gravitational-wave instrument and will listen to frequencies between these two regimes: the viola and cello sections of the orchestra.”
LISA will be made of three spacecraft, each containing a solid cube of gold-platinum, with lasers spanning the distance between them to note any changes caused by gravitational waves. This concept requires mind-boggling precision: the spacecraft must be ultra-stable, ensuring only the movement of spacetime affects the test masses, to measure ripples of only a few billionths of a millimeter. The spacecraft will also each be separated by a length “larger than the diameter of the Sun,” says Barke.

This technology will allow astronomers to track the collisions of binary stars in the Milky Way, map the gravity around the most monstrous black holes lurking at the centers of galaxies, and test relativity in such an extreme environment that “we might see deviations from Einstein’s prediction,” says Barke. Scientists also hope LISA will help solve a long-standing question in astronomy: how do supermassive black holes grow to their gargantuan sizes? The upcoming experiment will finally be able to observe so-called intermediate-mass black holes, the “elusive middle-children of the black hole family” according to Zevin, which weigh in at thousands of times the mass of our sun and could be a key step on the path to becoming supermassive.
Sumeet Kulkarni, a gravitational wave astronomer at the University of Mississippi, also notes the gravity of this moment for those of us on Earth: “The confirmation that LISA will actually fly in the near future opens so many new research opportunities and challenges to overcome for an entire generation of physicists, astronomers, and engineers.”