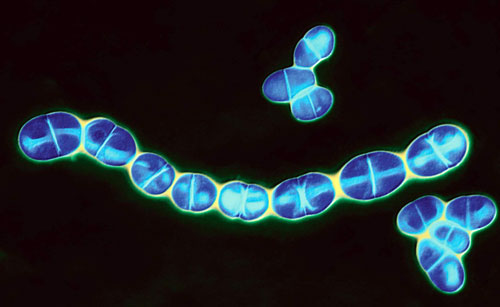
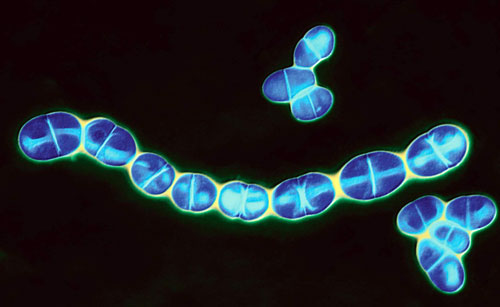
It’s a drizzly morning on New York’s Upper East Side, and Rockefeller University microbiologist David Thaler is sipping a double espresso amid the retro-hippie pillows and dangling paper stars of Java Girl, a favorite haunt of the neighborhood’s brainiac Nobel laureates, aging poets and famous entertainers. Thaler somehow manages to embody all three—a long, graying ponytail curling down the middle of his back, wire-frame glasses askew over expansive brown eyes, and a schnozz to rival an Einstein, Ginsberg or Allen. Thaler is one of the leading cheerleaders for a new field of biotechnology aimed at engineering the bacteria inside us to deliver drugs, destroy tumors, actively fight infection, and even vaccinate against their disease-causing kin.
Our ancestors, Thaler explains, emerged from the Stone Age by genetically engineering plants and animals through selective breeding, transforming the wolves that preyed on their flocks into the domestic dogs that would guard them. “Except for wild-caught fish, virtually everything we eat today has been engineered,” he says. “Meanwhile, we’re walking through this ocean of bacteria and only looking at them as something that can make us sick, rather than something to cultivate.” He believes that it’s time to move humanity from being microbe exterminators to microbe farmers.
Thaler thinks we need what he calls a “second Neolithic revolution.” Although his day job as a microbiologist at Rockefeller revolves around such abstract research as testing life’s speed limit (current record for replication: eight minutes), he sees himself as an idea man, someone who might help advance an entirely different mind-set in medical microbiology: Instead of using antibiotics to kill harmful bacteria in our bodies and our environment, why not coax bacteria to do our bidding?
“The technology to harness these bacteria exists,” Thaler says. Biotechnology firms already use bacteria like E. coli as tiny factories. Just slip the DNA instructions for, say, a new protein-based drug into E. coli and, in its endless quest to replicate itself, the bacterium will replicate the drug as well.
But it’s one thing to employ genetically engineered bacteria to produce pharmaceuticals inside a sealed vat. It’s quite another to deploy what some call “Frankenbugs” inside a patient. The same characteristics that make bacteria so amenable to genetic engineering—their malleability, their incredible replication speed, their genetic promiscuity—allow their newly acquired DNA to spread to other microbes, including potentially dangerous ones.
Such concerns have largely kept the first generation of engineered superbugs confined to biohazard-containment labs. But the few microbes that have made it into limited human trials—a cavity stopper, a tumor destroyer, a bowel soother—have been enticingly successful. And so the first standoff over body-ready bugs is taking place before the review boards of medical centers and government regulatory agencies, the people who will decide if the world is ready for engineered superbugs.
“I honestly think people are more comfortable with the idea of nano-robots scurrying through their bodies than they are of deploying bacteria,” Thaler muses. “But when you think about it, you cultivate your lawn. You’d probably like to cultivate your internal landscape.”

THE CAVITY KILLER
Jeffrey Hillman, an oral biologist for-merly of the University of Florida, is a poster child for the kind of biotherapeutic future that Thaler envisions. Hillman has spent a decade lobbying the FDA to let him test a transgenic tooth bug in volunteers. “Fortunately, we had no idea what was ahead,” says Hillman of the gantlet of regulatory requirements he has had to tackle since 1996. That was the year Hillman founded Oragenics, a biotech firm dedicated to commercializing his patented cavity-preventing Streptococcus mutans, a genetically modified organism (GMO) that’s the product of nearly 30 years of research.
Inside the mouth of most every person on the planet, colonies of S. mutans bacteria thrive on leftover sugars. The by-product of their digestion is the acid that eats away at tooth enamel and causes cavities. But there are many different strains of S. mutans, and some cause more trouble than others. In the summer of 1976, Hillman was trying to replace cavity-prone strains with those that secrete less enamel-eroding acid. Unfortunately, it seemed almost impossible to permanently eradicate a person’s “native” S. mutans once his or her teeth became colonized in early childhood.
“We were trying all sorts of crazy things,” Hillman recalls. “One time, we were painting volunteers’ teeth with iodine. Then we tried fitting their teeth with trays filled with antibiotics.” Yet no matter how thoroughly Hillman banished his volunteers’ native S. mutans or how quickly he re-colonized their teeth with a benign strain, the switch-out never stuck. “Slowly but surely, a person’s indigenous strain always came back,” Hillman says.
In 1982 Hillman hit on the idea of first finding a strain aggressive enough to elbow out a person’s native tooth tenants and then knocking out its genes for acid production. He conducted the microbial equivalent of cockfights, setting various strains of S. mutans against each other in crowded petri dishes. He knew he had found his ideal candidate when he saw that one “pinprick” colony had cleared a perfect circle in the lawn of other bacteria around it. When Hillman and two of his labmates introduced the strain into their own mouths, it quickly took over, banishing their native S. mutans in the process.
Next, Hillman deleted the microbe’s gene for acid production, but the superbugs didn’t survive the genetic tinkering. Most strains of S. mutans, including this one, use lactic acid to dispose of metabolic waste. Without acid excretion, the waste builds to toxic levels, killing the microbe.
Hillman solved the problem by making his bug produce alcohol instead of acid. To do so, he borrowed a gene for alcohol production from Zymomonas mobilis, which is used to make pulque, or Mexican beer. The resulting bug didn’t produce enough alcohol to make its host at all tipsy. But in studies with lab rats, it replaced the animals’ existing S. mutans and kept the rats mostly cavity-free on a high-sugar diet that would normally destroy their teeth.

The trouble was that Hillman now had a true transgenic—an organism that expressed the genes of two different species. The prospect of tests in humans meant that he had to go to the FDA for approval. The FDA eventually referred his case to the National Institutes of Health’s Recombinant DNA Advisory Committee, created in 1974 in response to public concern over the safety of interspecies gene transfer. The committee, which includes ethicists and patients as well as scientists and physicians, reviews any application for a transgenic intended to be used outside a sealed laboratory.
In 2004, the committee gave Hillman the green light. Usually, this is enough for full FDA approval. But not this time. FDA regulators asked Hillman to cripple his bug to guarantee that it could be removed should it ever cause problems. “When we asked them what kind of problems, they had no idea,” he recalls. “I guess we were setting a precedent.”
The regulators saw a genetically modified bacteria that was robust enough to take over any person’s mouth, and they were worried about its unchecked spread. Their decision reflected a common criticism of GMO biotherapeutics. “The main problem . . . is that [GMOs] are usually poorly contained,” argues geneticist Joe Cummins. Recently retired from the University of Western Ontario, Cummins is a leading spokesman for the London-based Institute for Science in Society, an anti-GMO lobbying group. “They’re bound to escape and to pollute the systems of people who don’t require therapy.”
So Hillman knocked out more genes, this time rendering his microbe unable to survive without an amino acid that test subjects would need to supply, twice daily, by rinsing with a specially formulated mouthwash. In addition, the agency required that Hillman test on patients wearing full dentures that could be dropped into bleach at the end of a week. The volunteers could not have children in their homes, and their spouses had to wear full dentures as well. And both the volunteers and their spouses had to be robustly healthy and under age 55. “We screened more than 1,000 potential volunteers,” Hillman says, “and we found two.”
The miniature, two-person trial proceeded without a hitch at the end of 2006, with no adverse side effects and complete elimination of the organism at the end of seven days. Last November, past the 10th anniversary of his original FDA application, Hillman received approval to use his crippled transgenic in a larger clinical trial. “Real people with real teeth!” he exults. For safety, the volunteers will spend the weeklong trial in a biocontainment ward.
Should his superbug prove as harmless as it appears, Hillman hopes the FDA will eventually allow him to skip the step where he renders it a nutritional cripple. Users could then dispense with the daily amino-acid mouthwash.
Might the bug then begin spreading from one person’s mouth to the next? It’s unlikely, Hillman says. When he and his labmates colonized their teeth with their GMO’s ancestor, it did not spread to wives and girlfriends, even while remaining in their own mouths for decades.
Proponents like Thaler ask whether such an “uncontrolled release,” if it were to occur, would be a bad thing. “What would it be like for us to have benign versions of Typhoid Mary walking around,” he asks, “spreading their health-enhancing germs?” In some cases, though, uncontrolled release of genetically modified bacteria could lead to disaster, even if the intended effects were nothing but beneficial.

ATTACKING CROHN’S
On day three of the study at the Academic Medical Centre in Amsterdam, the 43-year-old Dutch farmer felt so good that he was packing his bags to leave the hospital. The nurses caught him just as he was headed out the door of the center’s new biocontainment ward for gene therapy. Its rooms are kept under negative pressure so that even if a window breaks, bacteria-laden air will flow in, not out. The man had been spending his days confined to little more than a glorified hospital room, with doctors and nurses coming and going in head-to-toe surgical garb. The bug that was healing his body had to remain isolated, by government order. “We had to explain to him that he was not free to leave, no matter how wonderful he felt,” recalls study leader Maikel Peppelenbosch.
Over the previous eight months, Peppelenbosch had managed to win government approval for a clinical trial that deployed a genetically modified cheese-making bacterium, Lactococcus lactis-Thy12, to relieve Crohn’s disease [launch the gallery
here, to see how it works]. This excruciating bowel disorder is caused by the immune system mistakenly attacking the intestines’ normal complement of digestive microbes. The result is a vicious cycle of painful inflammation and gaping ulcers that can progress to life-threatening perforations of the colon.
Dutch approval of the trial—and the willingness of patients to cycle through 11 days of biological isolation—was a testament to both the seriousness of the disease and the lack of reliable cures, Peppelenbosch says. “These were patients for whom taking out the bowels was their last remaining option.” Funding for the study came from the U.S., by way of a private research grant from billionaires Eli and Edythe Broad, whose son suffers from Crohn’s.
The way to treat the disease is to turn off the immune system’s attack on the intestines’ native bacteria. Researchers have long known that lab animals whose bodies fail to produce the immune-calming molecule interleukin-10 develop severe inflammatory bowel disorders similar to Crohn’s. But efforts to administer IL-10 are fraught with problems. Stomach acid destroys the protein, so it can’t be taken by mouth. And introducing it into the bloodstream risks paralyzing a patient’s immune system.
Any solution must deliver the immune-calming molecule exactly where it’s needed—inside the intestinal tract—but nowhere else. That’s where Lothar Steidler’s creation comes in. In 1999 Steidler was pursuing postdoctoral studies into Crohn’s-disease treatments at Ghent University in Belgium. In an impressive molecular sleight of hand, Steidler took the gene for IL-10 and slipped it into L. lactis.
But he didn’t stick it just anywhere in the cheese bug’s genome. Steidler understood how important it was to prevent his bug from escaping into, say, the sewer system, where any number of nasty, disease-causing bacteria might pick up the IL-10 gene. The result could be pandemic disaster: a pathogen out in the wild with the ability to cripple the body’s disease-fighting response.
“I knew I had to build in some sort of suicidal mechanism,” he explains. He also had to prevent gene swapping between his “good bug” and a potential bad guy. So Steidler made sure that the incoming IL-10 gene always replaces another gene needed to produce the nutrient thymidine. That way, his new bugs can’t make thymidine, and so they die of nutrient starvation within a few days. That fleeting life span is enough to complete their mission but not long enough to survive in the waste that flushes down the toilet.
Even better, if the inserted gene jumps into another organism, it replaces that microbe’s thymidine gene. So any bug that receives the gene likewise becomes a doomed nutritional cripple. “Fortunately, Lothar designed this bacterium very well,” says Peppelenbosch, who collaborated with Steidler to usher the transgenic through regulatory approval in the Netherlands. Their proposal received no objections from either regulators or the public—an unexpected feat in rabidly anti-GMO Europe, he notes.
The team faced no lack of volunteers for the trial. The doctors at the Academic Medical Centre saw scores of patients with severe Crohn’s that failed to respond to standard anti-inflammatory drugs. The researchers ushered 10 patients into their containment ward, one by one, for their seven-day treatment and 11-day isolation.
Eight of the 10 Crohn’s patients experienced relief from pain and diarrhea, five dramatically so. One withdrew early for unrelated reasons, and none experienced any worsening of symptoms or problematic side effects. Most important for the prospect of larger studies, Steidler demonstrated that his transgenic microbe completely disappeared from the volunteers’ stool within a day of swallowing their last capsules of live bacteria.
As expected, the patients’ symptoms reappeared a few weeks after they returned home, and several came back to plead for continued treatment. “We couldn’t, of course,” Peppelenbosch says, because the trial was over. Steidler and Peppelenbosch are seeking Dutch approval for a larger, placebo-controlled trial, this time without the onerous restrictions of isolating patients on a biohazard ward.
Built-in suicide mechanisms such as Steidler’s may prove key to the widespread use of GMO biotherapeutics. “Now that the biocontainment issues are being fully recognized and achieved, I think it’s all going to move very quickly,” predicts North Carolina State University micro-biologist Todd Klaenhammer.
THE TUMOR BUGS
In January 2002, doctors at the Mary Crowley Medical Research Center in Dallas began injecting a genetically modified breed of salmonella into three cancer patients with large, inoperable tumors that had failed to respond to radiation or chemotherapy. For reasons still poorly understood, salmonella proliferates inside malignancies, perhaps because cancerous tumors tend to remain beyond the reach of the immune system. This salmonella was special, though. A Yale University team led by microbiologist David Bermudes inserted an E. coli gene into the bacteria. The gene produced an enzyme that activates a highly noxious, tissue-destroying drug. “The beauty is that neither the enzyme nor the drug that it activates does anything toxic except in places where they end up together,” Bermudes explains. In other words, the system is engineered to be harmless outside a tumor but deadly inside it.
The 2002 pilot trial proved a success, in that the bioengineered salmonella delivered its enzyme payload, produced a modest shrinkage in tumor size, and did no harm to the three patients, but the trial was too small to make any claims of a cure. To move into larger, meaningful trials would require following in Hillman’s footsteps through a battery of federal regulatory review boards. That costs money. Even if the researchers received approval to go ahead, they would need to come up with the many millions of dollars needed to usher any potential cancer treatment through large-scale patient trials.
That investment would most likely come from Vion Pharmaceuticals, the Connecticut biotech firm that currently holds Bermudes’s patent on the tumor-busting salmonella. Vion has no plans to tackle the regulatory process in the near future, however, says Ivan King, Vion’s vice president for research and development. “As a small company, we cannot move many things forward at any one time,” he says. What’s needed, he believes, is interest from a larger pharmaceutical company with much deeper pockets—just the kind of company that has yet to show interest in highly experimental bioengineered bacteria.

THE NATURAL WAY
Meanwhile, some researchers are focusing on unmodified microbes that could benefit the body. These “probiotics” are sold in grocery and health-food stores, yet few of the numerous available products have been rigorously tested. One of the exceptions is Lactobacillus GG, or “Culturelle,” isolated in the 1980s by Sherwood Gorbach and Barry Goldin of Tufts University. Over the past two decades, Gorbach, Goldin and others have published 250 scientific papers on this strain’s disease-fighting effects. Studies suggest that the bug has an immune-calming effect that may ease some food allergies. But its one clear and proven benefit is to reduce a person’s risk of picking up one of the many nasty intestinal bugs that cause food poisoning, traveler’s diarrhea and antibiotic-induced gastroenteritis, which results when antibiotics kill off a person’s normal intestinal bacteria and a disease-causing invader moves in.
In Europe, where probiotics have long been popular, they have also been used to prevent chronic respiratory and ear infections. In the early 1990s, Swedish ear-nose-and-throat specialist Kristian Roos developed a throat spray containing a medley of throat bacteria that dramatically reduced the recurrence of chronic strep infections. A few years later, Roos developed a similar concoction that protected toddlers and preschoolers who were predisposed to ear infections.
Roos’s probiotics demonstrated their worth in small clinical trials. But they also illustrate the challenge of developing a natural probiotic into a medical therapeutic. A small clinical trial may be enough to put a health claim on a nutritional supplement sold over the counter. But Roos wants to see such cures in the hands of doctors, who would judiciously prescribe them to patients. To do that, he must prove that his probiotics work in the same kind of large, multimillion-dollar trials that have stymied Bermudes’s cancer-fighting GMO.
For that kind of money, Roos admits, investors are right to expect an ironclad patent to protect their investment. But that’s difficult to do with bacteria that occur naturally on and in the human body. “Even though we can patent our particular mixture of organisms, it would be easy for someone else to come along and put together something slightly different from the hundreds of protective strains found in people’s throats,” he explains. Without the assurance of some meaningful patent protection on his product, he has been unable to attract financial investors, and his treatments languish in a storage freezer.

THE GOOD-MOOD BUG
Microbiologist John Stanford of University College London and his wife, Cynthia, discovered Mycobacterium vaccae while searching for a tuberculosis vaccine booster in Uganda in the early 1970s. Experts had long proposed that the widely variable efficacy of the TB vaccine stemmed from bacteria in a region’s soil that provided a natural booster effect. The Stanfords, crisscrossing the African nation in search of this bacterium, isolated M. vaccae, a benign genetic cousin of Mycobacterium tuberculosis, from the muddy shores of Lake Kyoga, an area where the TB vaccine proved unusually effective against both tuberculosis and leprosy. The Stanfords hoped that injections of M. vaccae would help prevent or cure TB, but at best their vaccine proved only mildly beneficial. More curious were anecdotal reports of unexpected benefits—regressions of allergies, asthma and even cancer.
In 1992 John Stanford and his colleague Graham Rook went on to form a publicly traded company, SR Pharma, to test these immune-boosting benefits in clinical trials with late-stage lung-cancer patients. But in 2001, under a spotlight of media attention, the trial failed to appreciably increase patients’ survival time. SR Pharma’s stock crashed, and following a dispute over the company’s future focus, the company removed Rook and Stanford from its board of directors.
Yet the trial did produce one bona fide benefit: a significant increase in “quality of life” among patients who got M. vaccae injections versus those who received a placebo. That dovetails with the work of University of Colorado neuroscientist Christopher Lowry, who last May published a study where he used M. vaccae in psychotropic experiments with rats. Lowry discovered that the bug increased brain levels of the mood-enhancing hormone serotonin and decreased depressive behavior. Even more promising, Lowry showed that M. vaccae appeared to be more discriminating than antidepressant drugs in the kinds of brain neurons it activates. It switches on the serotonin neurons associated with enhancing mood, without stimulating those that increase hyperalertness—that is, anxiety and sleeplessness. “Prozac without the side effects,” he calls it. In addition, recent studies have shown that M. vaccae may be effective against TB—the Stanfords’ original studies didn’t supply enough doses—and may increase the survival times of some late-stage cancer patients.
It’s just this sort of surprising potential that inspires researchers. “We’re always saying things like, ‘I feel lousy today. I must have caught a bug,’ ” Thaler says. “We never say, ‘I feel great. I must have picked up an endorphin-producing one.’ What would it mean to cultivate yourself to be contagiously healthy?”
Jessica Snyder Sachs is a contributing editor at Popular Science_. Her most recent book is_ Good Germs, Bad Germs: Health and Survival in a Bacterial World.




