The Church Of George Church
From reviving extinct species to hunting for dark matter, can a single scientist transform biology--and our lives?
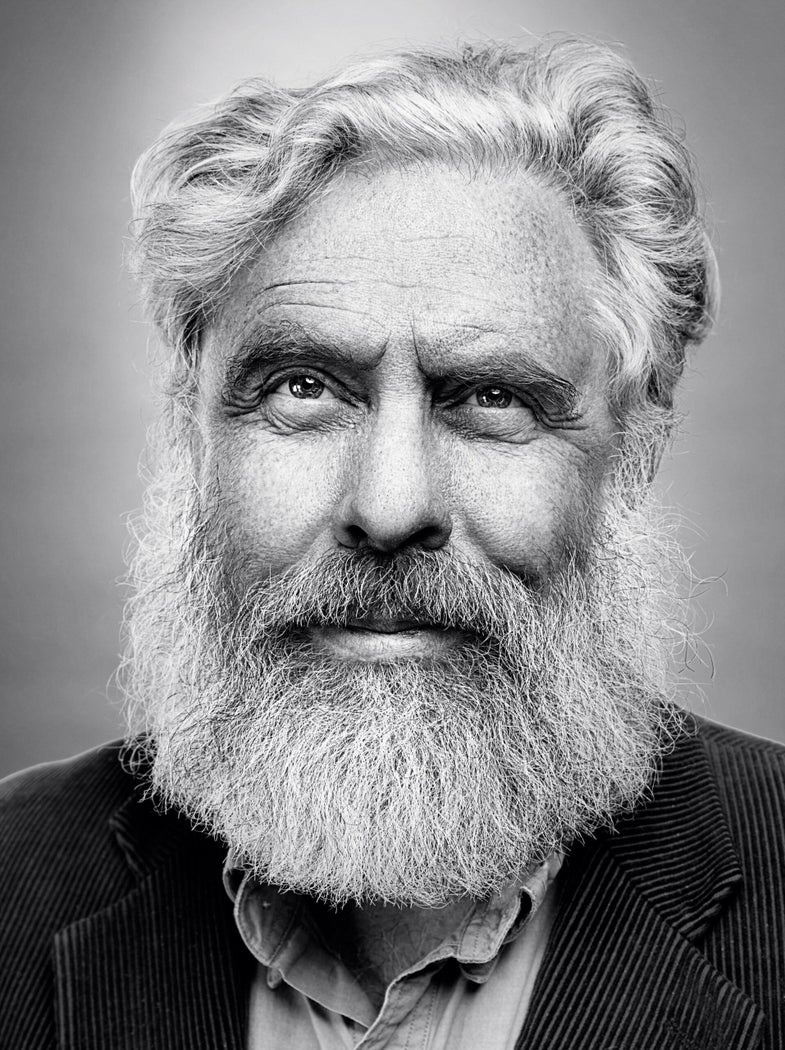
A few years ago, George Church was peer-reviewing a paper for the journal Science when he got an idea. The paper’s authors had encoded their names and a string of famous quotes into a bacterial genome to demonstrate the power and possibility of synthetic biology, the design and manufacture of DNA in a lab. Their encoding method was good. But Church, a genetics professor at Harvard Medical School, thought he could do better.
Sitting at his desk, in the space of a few minutes he wrote a computer program that translated his review from Roman letters into binary code and then into genetic code. He submitted the review to the journal’s editor, who couldn’t read it. The document contained only As, Ts, Cs, and Gs, shorthand for the nucleotides in DNA. The editor passed it along to the study’s authors. “At first, they had no idea what it said,” Church says, chuckling.
That should have been the end of it. The paper was published, and the stunt passed quickly into the stock of Church lore, in league with the time he lived for a year on nutrient broth from a lab vendor or the time he wore homemade blinders around the lab to make a statement about tunnel vision.
But Church wasn’t done. He wanted to see how far he could take his computer program, so he began looking for something else to code, something larger than a single review. He settled on his own book, Regenesis, which was about to be published. He spent a few days adapting the program he’d written and converted all 350 pages of text and photos into a string of As, Ts, Gs, and Cs. In the lab, he synthesized a strand of DNA to match the code, replicated it many times over, and dabbed a drop of the synthetic DNA onto a scrap of paper. The dot contained 70 billion copies of the book. It was no bigger than a period.
A few months later, Church took his experiment with him on The Colbert Report, pulling out the paper scrap–about the size and shape of a fortune cookie slip–for the world to see. Before he knew it, representatives of several companies tasked with archiving films and recordings were knocking on his office door.
“They said, ‘We want to do data storage,’ ” Church tells me. “I said, ‘You guys realize this is super academic, right? There’s no company or anything.’ And they said ‘Yeah sure, but data storage is a big problem.’ ”
With that, his parlor trick sprouted into a business. Members of Church’s lab are now scaling up the process in order to encode just about any form of media into DNA. If they succeed, they will have not only revolutionized data storage but ushered in what is perhaps biology’s most exciting era: the era when our capacity to manipulate the basic code of life dwarfs the capacity of even our most advanced computers.
Church tells the story pretty calmly, considering. We’re sitting in his office, a generous space tucked into the side pocket of a large lab, with windows that look down on Boston’s Longwood Avenue. He’s leaning back in his too-small office chair, stretching his legs out in front of him (he’s six-foot-five) and occasionally tugging at his Darwin-esque beard. He has blue eyes, a kind face, and an even voice, none of which betray the excitement or manic brilliance that one assumes must fuel such breakthroughs. If anything, he seems entertained. This is an amusing anecdote. The rest is an aside.
Church is helping to forge a new kind of biology, one less geared toward studying DNA than harnessing it for our own aims.
Church has already transformed biology once before. The DNA-sequencing technology he first devised in graduate school helped reduce the cost of whole-genome sequencing from billions of dollars to thousands. Scientists are now using it to investigate intractable diseases, such as cancer and schizophrenia, and doctors are beginning to use it to identify genetic mutations that cause rare–and, until now, undiagnosable–illnesses.
Amid the bays and bench tops that constitute Church’s laboratory, a litany of other revolutions are taking shape: His team of researchers is coaxing the woolly mammoth back from extinction by melding its genome to an elephant’s. They’re devising ways to inoculate mosquitoes (and, by extension, us) against malaria. And they’re designing tools to help physicists hunt down dark matter and to help neuroscientists map the human brain. Church has launched 15 bioengineering start-ups in the past decade and advises a number of others. More so than any other scientist in his field, he is helping to forge a new kind of biology, one less geared toward studying DNA than harnessing it for our own aims.
In the book that he encoded in DNA (which, incidentally, is also available printed and bound), he and his co-author, Ed Regis, envisioned the future this new biology could bring, one in which bacteria fuels cars and commercial jets and humans are immune to cancer. It may sound like science fiction, or at least like a litany of overhyped pipe dreams that science so often sells. But George Church’s pipe dreams have an uncanny record of becoming reality.
•••
For all his eccentricities, Church is an easy person to talk with. And yet such is his reputation for brilliance that people seem always to be apologizing to him for their own intellectual shortcomings. “I’m sorry if this sounds stupid,” people say. I caught a reporter doing it, and a CEO. Then, I did it too.
In fact, on my first visit to his office, I manage to completely embarrass myself. It’s early afternoon, and he’s invited me to sit in on a telephone interview with a radio reporter, before I tag along to some meetings. A handful of 3D-printed molecules are scattered across the big round table in the center of his office. He has been fidgeting with one–absentmindedly pulling it apart, between his thumb and forefingers before letting it snap back together–while he leans back in his chair and talks at the speakerphone. I pick up another molecule, one that looks identical to his but is not. Instead of snapping back together when I pull at it, it explodes.
Twelve plastic pentagons and one large white die go flying through the air. They skittle loudly across the table and roll onto the floor. Some of them land under Church’s desk. I am mortified. I scramble to gather all the pieces and reassemble them as quietly as possible, while he calmly continues to answer questions about genomic medicine.
When the phone call ends, I make sheepish apologies. “I thought by the way yours was snapping back together …” I say, trailing off into the ether.
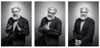
George Church
Unfazed, he takes the two molecules, holds them side by side, and launches into a geometry lesson. They are both dodecadedrons, he explains–objects with 12 sides. He has rigged his with some putty to prevent the pieces from disassembling; mine has not been so rigged. It is also wrapped around a 20-sided die, or an icosahedron. “I found this die on the street,” he says. “I like it because it looks like the nuclear material of a virus.” He goes on to explain the significance of these shapes: Icosahedrons are among the most symmetrical structures in the natural world, he says. They also form the basis of viral symmetry. I pretend to understand, but my takeaway is simpler than he probably intends: George Church is the kind of guy who finds a 20-sided die and immediately thinks about the structure of viruses.
He has been tinkering like this–testing the ways in which the world around him does and does not fit together–for most of his life. When he was 10 years old, he began replicating the work of a botanist named Luther Burbank by grafting the branches of one fruit tree onto another in his backyard. As a teenager at Andover, a private school, he taught himself BASIC computer programming and then taught the school’s computer linear algebra. As an undergraduate at Duke University, he raced through the curriculum and simultaneously completed two bachelor’s degrees (in zoology and chemistry) in two years. As a graduate student there, he helped solve the structure of transfer RNA, which translates the nucleic acids of DNA into the amino acids of proteins. He spent so much time in the lab that Duke expelled him from its Ph.D. program for missing too many classes. Fortunately, Harvard took him in.
“It was pretty clear he was a different sort of bird right from the beginning,” says Gary Ruvkun, a Harvard geneticist and good friend of Church’s (the two attended graduate school together). “I still remember I would work late, and when I’d be leaving the lab at, like, 2 a.m., he’d be buzzing in on his bike, ready to start the day.”
It was at Harvard that Church met his future wife, a fellow graduate student named Ting Wu. He followed her across the country and back before the couple settled in Boston to grow their labs (both at Harvard) and their family (they have one daughter, now in her 20s). His wife, he says, is a much better geneticist than he. In fact, Church is fairly adamant that he’s not a scientist at all but an engineer who occasionally does some science.
Like an engineer, he tends to see the universe not as a disparate set of mysteries but as a machine with a vast array of buttons and levers, each begging to be pushed and pulled. “His approach to science is modeled after the home-brew computer clubs, where the computer revolution was started in basements and garages,” Ruvkun says. “He told me once that he’s more proud of his National Academy of Engineering membership than of his National Academy of Sciences one. Nobody else I know thinks that way.”
•••
Here’s how it goes in most academic laboratories: A scientist develops proficiency in a handful of techniques and uses those techniques to study a handful of closely related questions. That lab attracts students and junior researchers with narrowly aligned interests and experiences. The entire system tends toward specialization, so that, by and large, molecular biologists interested in immunology and experienced with mice go to one kind of lab and neuroscientists interested in the visual system and experienced with flies go to another. Expertise is cultivated and refined and, in some cases, hoarded like wartime food rations.
Church’s lab is the opposite. Rather than seeking homogeneity, he recruits as diverse a group as possible. Physicists and neuroscientists work alongside geneticists, engineers, and entrepreneurs. “The image for me is of poking deep holes all over the place, in the fabric of science and engineering,” he says. “As we probe each of those points, we get cross talk.”
“The World Wide Web went from zero to millions of pages in a few years. Many revolutions look irrelevant before they change everything.”
The result is that his lab manages to be both one of Harvard’s top producers and a well-known receiving center for science’s misfit toys. There’s an artist encoding Wikipedia entries into apple genomes (to create a literal tree of knowledge) and an insurance industry refugee who fled his office job over a decade ago, worked several months for free while teaching himself biochemistry, and now serves as “co-head” of the lab. For a time, there was also a passenger-pigeon obsessive who had no advanced degree but had heard about Church’s mammoth de-extinction project and cold-called asking to participate; he has since gone on to lead the pigeon resurrection initiative out of a lab in California.
“We always joke that the only thing you need to do to join George’s lab is show up,” says Uri Laserson, a former student of Church’s and the co-founder of Good Start Genetics, a company that offers genetic screening for inherited diseases. “There is zero organization. His style is just to let things happen.” It’s a scrappy kind of place, Laserson says–a little bit sink-or-swim–but not in a bad way. “Mostly, you have the constant sense that exciting things are happening or are about to happen and if you miss out on it, you have only yourself to blame.”
The projects that emerge from this controlled chaos seem to fall along a continuum. At one end, the lab applies biology to very specific non-biological problems. Data storage is an example, but lately, members of Church’s lab have been using DNA to help physicists track dark matter, a mysterious substance that makes up some 27 percent of the known universe. At the other end of the continuum, Church’s team employs the tools of other sciences to solve the problems of biology. For example, the newly developed technique FISSEQ (fluorescent in situ sequencing) draws on a few different subsets of physics to help geneticists visualize gene expression in living cells. Before FISSEQ, scientists could measure just three or four genes at a time; now, they can measure thousands at once.
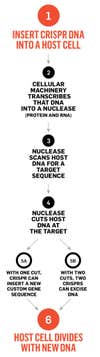
Genome Editing In Six Easy Steps
But it’s the projects in the middle of the continuum–biology-based solutions for biology-based problems–that tend to garner the most attention. Many of these involve CRISPR, a genome-editing tool derived from bacteria that uses an enzyme to excise specific nucleotide sequences and swap them with others. With it, scientists can alter multiple genes at once, without having to do the arduous work of extracting, isolating, and cloning the original genetic material and cross-breeding the resulting transgenic animals. A few other tools offer a similar shortcut, but CRISPR is much quicker. “It’s the fastest thing I’ve seen yet,” Church says. “It’s like you throw a piston into a car and it finds its way to the right place and swaps out with one of the other pistons–while the motor’s running.”
In January 2013, Church was one of the first to show that CRISPR could splice DNA in human cells. Since then, scientists have used it to correct a number of genetic problems, including certain forms of liver disease (in mice) and antibiotic resistance.
Church says CRISPR can address ecosystem-level issues as well. By circumventing the rules of sexual selection with live genome editing, CRISPR greatly increases the odds that a given gene will be passed from parent to offspring. The result is what scientists call a gene drive: That is, a gene is driven quickly through an entire wild population. Imagine that you insert a gene for malaria-resistance into a single mosquito and release that mosquito into the wild. You may eventually wipe out the disease altogether. Or imagine inserting a gene that renders an invasive species sterile. You could potentially rid an ecosystem of that species within a few generations.
CRISPR is also a key component of what may be the most high-profile of Church’s projects: de-extinction. When Stewart Brand, editor of the famed Whole Earth Catalog, hatched a plan to revive extinct species such as the woolly mammoth and the passenger pigeon, he turned to Church for the technology. Church devised an automated genome-engineering process (called the “evolution machine” by some) that enables researchers to meld together genomes from different species. His lab is now at work on a “cold-resistant elephant”–an elephant that has borrowed genes from the woolly mammoth so that it can thrive in colder environments. It could be possible to resurrect other species using the same approach, Church says. In fact, the possibilities are as limitless as our imaginations.
Whether that’s a promise or a peril depends on whose imagination we’re talking about.
•••
Before Church broke out the 70 billion copies of his book on The Colbert Report, the comedian asked him a question he gets a lot. “How do you think your work,” Colbert asked, “will eventually destroy all mankind?” The audience laughed riotously. “Do you think it’s going to be like a killer virus,” he asked, pausing to lean in and tap his fingers on the table. “Or more like a giant, mutant, killer squid man, who arises from the Pacific, between Easter Island and Chile, and feasts on our flesh?” Although it was a joke, the bit underscored a paradox that Church often faces in response to his work. On one side, there are skeptics who don’t believe the possibilities Church is peddling. On the other side, there are terrified believers who worry that it may be all too possible. Often, he alone stands in the middle.
Church tends to view this paradox through the same lens that he views most everything: as an engineering challenge. Take gene drives, for an example. Terrified believers worry about what could happen if a given gene drive has unintended effects. Say scientists introduce a gene that makes mosquitoes resistant to malaria, but that in turn causes an unexpected crash in the mosquito population that throws the ecosystem out of whack. Church’s response to such a disaster would be simply to deploy a reverse drive to try to undo the damage. Skeptics have a different set of concerns, namely that natural selection would just weed out the new gene over time, making gene drives unworthy of the effort and risk. To this, Church just says we could deploy the same drive periodically, making slight tweaks here and there.
To play either of these scenarios forward is to see the world through Church’s eyes: a place where DNA is the ultimate computer code and we are all computer programmers.
Of course, not all concerns are technical. Animal rights activists worry about a surge in animal experimentation now that CRISPR has made it easier than ever to create, for example, genetically modified monkeys. And corporate watchdogs have their own concerns about placing control of the human genome in the hands of private for-profit companies. It bears stating that Church works with a number of corporate sponsors, including Chevron, Procter & Gamble, and Merck.
In Church’s eyes, the world is a place where DNA is the ultimate computer code and we are all computer programmers.
Church makes no apologies for his entrepreneurship. He nearly always opens his lectures with a slide that displays all the logos of the companies he’s affiliated with. He agrees that there are risks involved in the work he does, but he does not think that vilifying corporations is the answer. “Industry is an essential part of what we do,” he says. “You can’t just hoard your ideas inside the ivory tower. You have to get them out into the world.”
Still, he takes safety concerns seriously. Last summer, Church and a few colleagues published two papers and one blog post on the same day, introducing the concept of gene drives and calling for “informed public discussion, regulatory review, and the establishment of guidelines for the safe development of the technology.” No one had actually made a gene drive yet, but Church wanted to get the ball rolling. “The important thing is to actually listen to the public’s concerns,” he says. “And then try to visualize things that can go wrong and think of ways to guard against them.”
To that end, he is devising new methods of biocontainment; the term normally refers to the physical safety measures (cabinets, hoods, isolation rooms) that laboratories use to prevent the escape of potentially dangerous organisms. In January, Church and members of his lab reported in the journal Nature that instead of relying on physical containment, they had managed to install protective measures within the microbial genome itself. They engineered a strain of bacteria that survives on a synthetic amino acid found only in the laboratory, not in nature.
And that’s how Church the engineer works. No obstacle is insurmountable. Technology moves toward greater efficiency and capacity, he says, not the reverse. And in any case, doubters come with the territory. “The World Wide Web went from zero to millions of web pages in a few years,” Church says. “Many revolutions look irrelevant just before they change everything swiftly.”
•••
The last time I chat with Church he is on a cellphone, walking from his office to a meeting down the street. The connection’s crackly, so I ask whether he’d prefer to talk later. “I like talking and walking,” he says. “It’s more productive than doing just one.”
I ask for an update, and he rattles off a string of developments. The company funding his data-storage project is planning to make a big announcement about his team’s latest advances. “We’ve scaled way up from the five megabyte book,” he says. He’s got a paper due out in the Proceedings of the National Academy of Sciences about the DNA and dark matter project. His group has also completed the first successful gene drive, a small pilot study in yeast that proved that foreign genes introduced to cells in a lab can be passed on to–and spread throughout–a separate wild population.
I ask him which project he’s most excited about. He answers without hesitation: CRISPR. “I like exponential fields,” he says. “Right now, nothing is more exponential than that.”
Church has helped launch Editas Medicine, a biotech company aimed at harnessing CRISPR’s therapeutic potential. The idea is to develop a new class of CRISPR-based drugs that can “surgically repair” aberrant genes. Editas has plenty of financial and scientific backing, but it’s still in early days. More than most, Church knows that ideas can often be ahead of their time. On more than one occasion, he has had to shelve a project–low-cost gene sequencing, for one–until the rest of the world was ready for it. “People think it’s great to be ahead of your time,” he says. “But it can actually be quite painful.” We should all be grateful that’s never stopped him yet.
This article was originally published in the May 2015 issue of Popular Science.